The sliding filament theory is a fundamental concept in muscle physiology that explains how muscles contract to generate force. This theory is based on the interactions between two types of protein filaments—actin (thin filaments) and myosin (thick filaments)—within the muscle fibers. By understanding this theory, we gain insight into how muscles function at a molecular level to produce movement and force.
Structure of Skeletal Muscle
Skeletal muscle fibers, also known as myofibers, are long, cylindrical cells that are multinucleated due to the fusion of precursor muscle cells during development. These fibers are specialized for contraction and contain several key structures:
- Sarcoplasmic Reticulum (SR): A specialized type of smooth endoplasmic reticulum that stores and releases calcium ions (Ca²⁺), which are crucial for muscle contraction.
- Myofibrils: These are the contractile elements of the muscle fiber, consisting of thick myosin filaments and thin actin filaments arranged in a repeating pattern along the length of the muscle fiber.
- T-Tubules (Transverse Tubules): Extensions of the sarcolemma (muscle cell membrane) that penetrate into the muscle fiber, allowing the action potential to reach the SR.
- Mitochondria: Numerous in muscle fibers to provide the ATP needed for contraction.
Structure of the Sarcomere
A sarcomere is characterized by its highly organized structure, which can be observed under a light microscope as a repeating unit of alternating dark and light bands. The key structural elements of the sarcomere include:
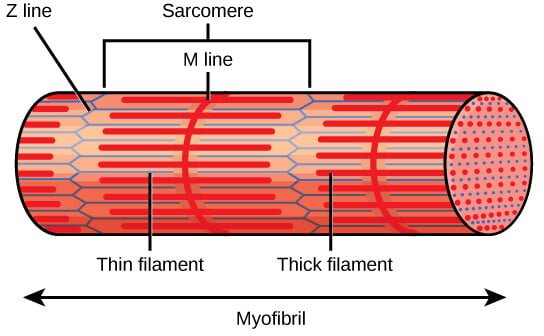
- Z Discs (Z Lines): The Z discs define the boundaries of each sarcomere and anchor the thin filaments of actin. They are located at both ends of the sarcomere and play a crucial role in maintaining the structural integrity of the sarcomere. The Z disc also serves as an attachment site for titin, a large protein that contributes to sarcomere elasticity.
- A Band: The A band is the region of the sarcomere that contains the entire length of the thick filaments (myosin). It appears darker under the microscope due to the overlap of myosin with actin filaments. The A band does not change in length during muscle contraction, as it represents the length of the myosin filaments, which do not shorten but rather slide past the actin filaments.
- I Band: The I band is the lighter region of the sarcomere that contains only thin filaments (actin) and extends from the end of one A band to the beginning of the next A band. The I band shortens during muscle contraction as the actin filaments slide inward, overlapping more with the myosin filaments.
- H Zone: The H zone is a central region within the A band where only thick filaments are present, with no overlap from thin filaments. It appears lighter than the rest of the A band. During contraction, the H zone narrows as the actin filaments slide into the region, reducing the distance between adjacent myosin filaments.
- M Line: The M line is a structural protein assembly located in the center of the sarcomere, within the A band. It helps anchor the thick filaments and maintains their alignment during contraction. The M line consists of myomesin and other proteins that stabilize the myosin filaments.
Myosin: Structure, Synthesis, Classification, and Functions – The Science Notes
Actin: Structure, Function, and Dynamics – The Science Notes
The Sliding Filament Theory
The sliding filament theory, initially proposed by A. F. Huxley and R. Niedergerke and later expanded by H. E. Huxley and J. Hanson, explains how muscle contraction occurs. According to the theory:
- Sarcomere Structure During Contraction: As a muscle contracts, the A band, which contains myosin filaments, remains the same length, while the I band, which contains actin filaments, shortens. This shortening is due to the sliding of actin filaments past myosin filaments.
- Actin-Myosin Interaction: Myosin heads bind to actin filaments, forming cross-bridges. These cross-bridges undergo a power stroke powered by ATP hydrolysis, pulling the actin filaments towards the center of the sarcomere and causing contraction.
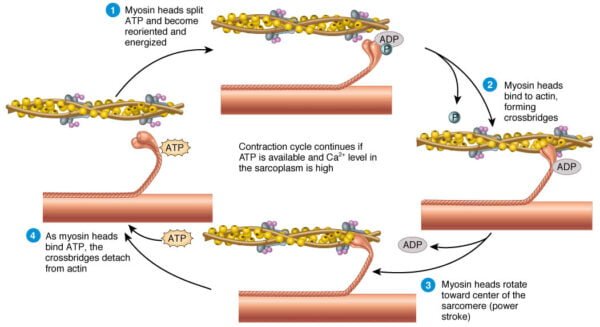
Steps of Sliding Filament Theory
According to the sliding filament theory, muscle contraction occurs through the sliding of actin and myosin filaments past one another, resulting in the shortening of the sarcomere without changing the length of the filaments themselves. The process involves several key steps:
1. Action Potential and Calcium Release:
- An action potential from a motor neuron arrives at the neuromuscular junction, causing the release of acetylcholine (ACh).
- ACh binds to receptors on the sarcolemma, leading to depolarization and the initiation of an action potential that travels along the T-tubules.
- The action potential stimulates the SR to release Ca²⁺ into the sarcoplasm.
2. Binding of Calcium to Troponin:
- Calcium ions bind to troponin C, a component of the troponin complex associated with the thin filaments.
- This binding causes a conformational change in the troponin-tropomyosin complex, moving tropomyosin away from the actin-binding sites.
3. Cross-Bridge Formation:
With the binding sites exposed, the high-energy myosin heads attach to actin, forming cross-bridges.
ATP bound to myosin is hydrolyzed to ADP and inorganic phosphate, providing energy for the power stroke.
4. Power Stroke and Filament Sliding:
- During the power stroke, the myosin head pivots, pulling the actin filaments toward the M line and shortening the sarcomere.
- The release of ADP and phosphate from the myosin head is followed by the binding of a new ATP molecule, which causes the detachment of the myosin head from actin.
5. Reactivation of Myosin Heads:
- ATP is hydrolyzed to ADP and phosphate, which re-energizes the myosin head, preparing it for the next cycle of binding and pulling.
- The cycle repeats as long as calcium ions remain present in the sarcoplasm.
6. Muscle Relaxation:
- When the action potential ceases, calcium ions are actively transported back into the SR.
- The decrease in calcium concentration causes tropomyosin to cover the actin-binding sites again, preventing further cross-bridge formation and resulting in muscle relaxation.
Regulation of Muscle Contraction
Muscle contraction is tightly regulated by several factors, including calcium ions and ATP:
- Calcium’s Role: Calcium ions play a pivotal role in muscle contraction by binding to troponin, a regulatory protein associated with actin filaments. In the absence of calcium, tropomyosin covers the myosin-binding sites on actin, preventing cross-bridge formation. When calcium levels increase, troponin undergoes a conformational change that moves tropomyosin away from the binding sites, allowing myosin heads to attach to actin.
- ATP’s Role: ATP is essential for muscle contraction as it provides the energy needed for the power stroke and the detachment of myosin from actin. ATP hydrolysis also allows the myosin head to return to its cocked position. Without sufficient ATP, muscles cannot relax and remain in a state of contraction, leading to conditions such as rigor mortis.
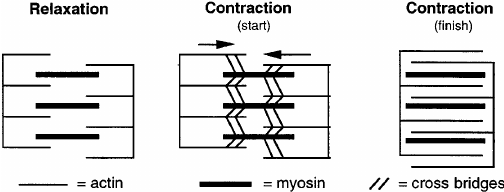
Detailed Mechanisms and Observations
- Molecular Mechanisms: The detailed molecular mechanisms of the sliding filament theory have been elucidated through various studies. For example, high-resolution microscopy and X-ray diffraction studies have provided insights into the structural changes that occur during muscle contraction. These studies have confirmed that the sliding of actin filaments past myosin is responsible for sarcomere shortening.
- Protein Structures: The structural details of the myosin and actin filaments have been further explored through techniques such as cryo-electron tomography and atomic force microscopy. These studies have revealed the precise arrangements of actin and myosin, as well as the conformational changes that occur during the cross-bridge cycle.
Evidence Supporting the Theory
Several observations support the sliding filament theory:
- Sarcomere Shortening: During muscle contraction, the distance between Z-discs decreases, the H zone narrows, and the I band shortens, while the A band remains unchanged.
- Microscopic Studies: Electron microscopy reveals that the actin and myosin filaments do not change length but slide past each other.
Conclusion
The sliding filament theory has profoundly shaped our understanding of muscle contraction, revealing the intricate molecular interactions that enable muscle fibers to shorten and generate force. By elucidating the roles of actin, myosin, ATP, and calcium, scientists have gained valuable insights into muscle function and regulation. Despite its comprehensive nature, ongoing research continues to refine our understanding and address unresolved questions. The continued exploration of muscle physiology promises to uncover new insights and applications, advancing our knowledge of this fundamental physiological process.
References and Further Reading
- Huxley, A. F., & Niedergerke, R. (1954). Structural changes in muscle during contraction: Interference microscopy of living muscle fibres. Nature, 173, 971-973.
- Huxley, H. E., & Hanson, J. (1954). Changes in the cross-striations of muscle during contraction and stretch and their structural interpretation. Nature, 173, 973-976.
- Spudich, J. A. (2001). The myosin swinging cross-bridge model. Nature Reviews Molecular Cell Biology, 2, 387-392.
- Lehman, W., Craig, R., & Vibert, P. (1994). Ca2+-induced tropomyosin movement in Limulus thin filaments revealed by three-dimensional reconstruction. Nature, 368, 65-67.
- Goody, R. S. (2003). The missing link in the muscle cross-bridge cycle. Nature Structural Biology, 10, 773-775.