Introduction to Actin
Actin, a critical protein in eukaryotic cells, serves as a fundamental component of the cytoskeleton, which provides structural support and shape to cells. It plays a vital role in numerous cellular processes including motility, division, and intracellular transport. The actin protein, with a molecular weight of approximately 43 kDa, is highly conserved across various species, emphasizing its evolutionary significance. There are three primary isotypes of actin: α-actin, β-actin, and γ-actin. Although these isotypes share over 90% amino acid homology between them and more than 98% within a specific isotype group, differences primarily occur in the amino-terminal 30 residues. This region of the protein is situated on the periphery of the actin double-helix in F-actin and is crucial for interactions with other proteins, including myosin.
Isotype Variations and Cellular Behavior
Actin isotypes exhibit distinct behaviors both in vitro and in vivo, reflecting their specialized functions within different cellular contexts. For instance, γ-actin has been observed to localize differently compared to other isotypes, indicating that the various isotypes of actin perform unique roles within the cell. This specificity is underscored by the differential binding of actin-associated proteins to each isotype. An illustrative example is the protein ezrin, which shows a particular affinity for β-actin, highlighting the importance of using the appropriate actin isotype in experimental setups to accurately replicate physiological conditions.
Recent studies have demonstrated that isotype-specific interactions of actin-binding proteins (ABPs) can significantly influence cellular processes. For instance, the differential subcellular localization of γ-actin suggests that specific isotypes are involved in distinct cellular functions, which could impact the interpretation of experimental results if the incorrect actin isotype is used.
Polymerization of Actin: From G-Actin to F-Actin
Actin exists in two main forms: globular actin (G-actin) and filamentous actin (F-actin). G-actin is the monomeric, soluble form of the protein, while F-actin refers to the polymerized, filamentous structure.
Polymerization Process: G-actin polymerizes to form F-actin under physiological conditions, driven by the hydrolysis of ATP. F-actin is characterized by a double-helical structure, which imparts intrinsic polarity to the filament. The plus-end (or barbed-end) of the filament exhibits a higher rate of polymerization compared to the minus-end (or pointed-end), creating an inherent asymmetry in the filament structure.
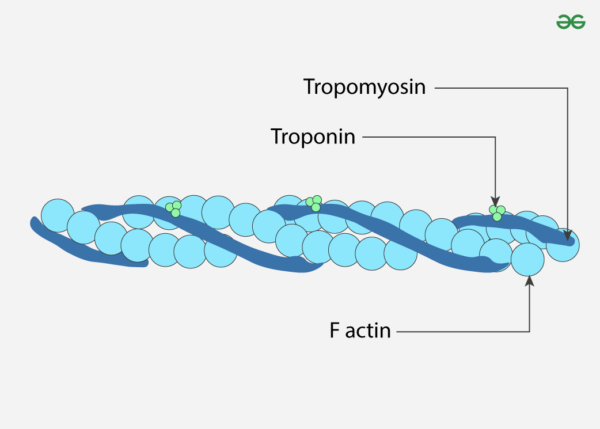
Critical Concentration (CC): The process of actin polymerization is regulated by the concentration of actin monomers. The Critical Concentration (CC) is defined as the monomer concentration at which the rates of polymerization and depolymerization are balanced. At concentrations above the CC, actin will polymerize until the free monomer concentration is reduced to the CC. For muscle actin at 4°C, the CC is approximately 0.03 mg/ml in the presence of 2 mM Mg²⁺ and 50 mM KCl. In the absence of these ions, the CC increases significantly, demonstrating how ionic conditions influence polymerization. Similarly, non-muscle actin exhibits varying CC values depending on the ionic environment and temperature, reflecting its dynamic nature.
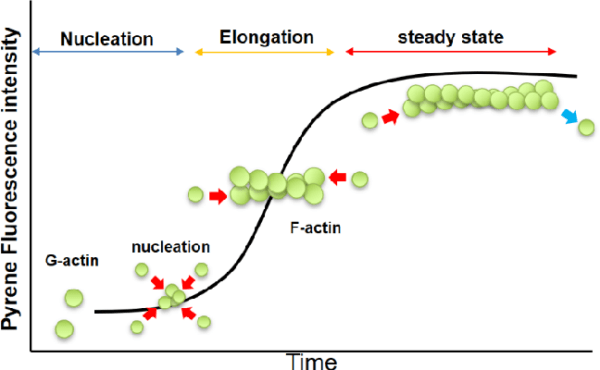
Measuring Actin Polymerization
Several methods are employed to measure actin polymerization, each with specific advantages and limitations:
- Fluorescence Enhancement of Pyrene Conjugates: This technique involves using pyrene-conjugated actin, which exhibits increased fluorescence upon polymerization. The fluorescence enhancement is up to twenty-fold, providing a sensitive and versatile method for monitoring actin polymerization in real-time. This method is advantageous for studying polymerization dynamics with minimal sample disruption.
- DNase Inhibition Assays: This assay exploits the high-affinity interaction between G-actin and DNase I. G-actin inhibits DNase activity, allowing for the differentiation between G-actin and F-actin. This assay is particularly useful for quantifying the amounts of monomeric and filamentous actin in cell extracts and studying actin-binding proteins that generate G-actin from actin filaments.
- Viscosity Measurements: Viscosity measurements can be performed using high or low shear methods, depending on the filament length. High shear methods are suitable for detecting small differences between short and medium-length filaments, while low shear methods are used for longer filaments. Although these methods can disturb the filaments and may not be highly accurate, they are useful for comparing filament cross-linking between samples.
- Spin-Down Assays: Spin-down assays rely on differential sedimentation to separate F-actin from G-actin. This method provides quantitative data on actin polymerization at steady state. It can be used in combination with other methods to quantify actin polymer over time. However, this method is destructive and may overestimate the amount of actin monomer due to incomplete sedimentation of small actin oligomers.
Modified Actins and Their Uses
Modified actins, such as fluorescently labeled or biotinylated actins, are valuable tools in research:
- Fluorescent Actin: Fluorescently labeled actins are used to study actin dynamics both in vivo and in vitro. For instance, fluorescent actin can be microinjected into cells to observe actin treadmilling or myosin mobility. This approach is useful for understanding the behavior of actin filaments in various cellular contexts.
- Pyrene Actin: Pyrene-conjugated actin is employed to monitor actin polymerization. The increased fluorescence of pyrene actin upon polymerization provides insights into the dynamics of actin filament formation.
- Biotin Actin: Biotinylated actin has multiple applications, including probing actin dynamics and selectively purifying actin using streptavidin-coated beads. It can also be used as a probe for actin dynamics in cells when combined with streptavidin or avidin-coated gold particles.
Actin Binding Proteins (ABPs)
Actin interacts with a diverse array of proteins, known as actin-binding proteins (ABPs), which significantly influence its function. Over 150 ABPs have been identified, constituting approximately 25% of cellular proteins. These proteins modulate actin dynamics and play crucial roles in various cellular processes:
- Muscle Contraction: Actin filaments interact with myosin to facilitate muscle contraction. This interaction is essential for muscle function and movement.
- Cell Motility: Actin-driven movements are critical for cell migration, allowing cells to move and change shape in response to various signals.
- Cytokinesis: During cell division, actin filaments form the contractile ring that separates daughter cells, ensuring proper cell division.
- Cytoplasmic Streaming: Actin networks support the flow of cytoplasm within cells, contributing to various intracellular processes.
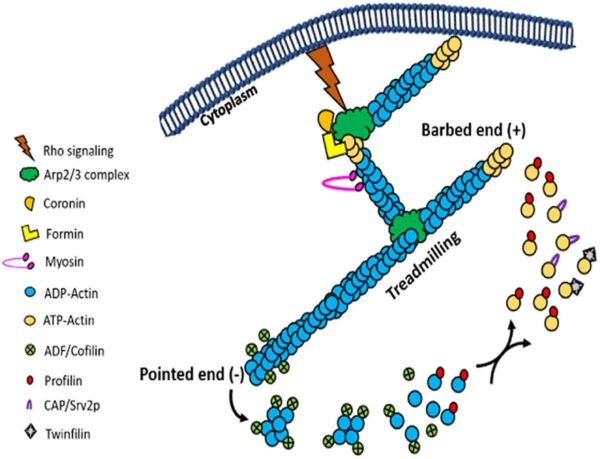
ABPs can be isolated and studied using a range of biochemical, genetic, and immunological techniques. Methods such as affinity chromatography and assays based on pyrene actin fluorescence are instrumental in identifying and characterizing these proteins.
Medical Significance of Actin Research
Actin research has profound medical implications, particularly in understanding diseases associated with cytoskeletal dysfunction. Some key areas of interest include:
- Erythrocyte Membrane Proteins: Mutations in proteins like spectrin, ankyrin, and band 3, which are involved in the erythrocyte membrane skeleton, lead to conditions such as hereditary spherocytosis and elliptocytosis. These mutations affect the shape and stability of red blood cells, resulting in increased fragility and lysis.
- Muscular Dystrophies: Dystrophin, an actin-associated protein, is essential for linking the actin cytoskeleton to the muscle cell membrane. Mutations in dystrophin result in Duchenne and Becker muscular dystrophies, characterized by progressive muscle weakness and degeneration. Understanding dystrophin’s role in muscle cells is crucial for developing therapeutic strategies for these conditions.
- Neurodegenerative Diseases: Proteins associated with the actin cytoskeleton are also involved in neurodegenerative diseases. For example, proteins that link the actin cytoskeleton to synaptic structures are critical for maintaining neuronal function. Dysregulation of these proteins can contribute to diseases such as Alzheimer’s disease and other neurodegenerative disorders.
Molecular Infrastructure and Dynamics
Actin forms a complex ultrastructure that supports cellular shape and motion. The cytoskeleton, composed of actin filaments, provides a scaffold for cellular organization and facilitates various cellular movements. Actin filaments are involved in the formation of pseudopods used by amoebas for crawling and the microvilli in intestinal cells, which extend into the digestive tract for nutrient absorption.
Actin’s dynamic nature is a hallmark of its function. Actin filaments are continually assembled and disassembled as the needs of the cell change. This dynamic behavior is regulated by ATP binding and hydrolysis. Free actin monomers bind ATP and associate with growing filaments. As ATP is hydrolyzed to ADP, the actin filament undergoes subtle structural changes, leading to a decreased stability and eventual disassembly. This dynamic process, known as treadmilling, involves continuous polymerization at one end and depolymerization at the other, allowing the filament to “move” through the cell without changing its overall length.
Controlled Growth of Actin Filaments
In cellular contexts, actin filament growth is tightly regulated to prevent uncontrolled polymerization. Proteins such as gelsolin and profilin play crucial roles in controlling actin dynamics. Gelsolin severs actin filaments, generating new ends for further polymerization, while profilin binds to actin monomers, promoting their incorporation into filaments. This regulation ensures that actin filament formation is responsive to cellular signals and needs, maintaining cellular integrity and function.
Future Directions in Actin Research
Advancements in actin research continue to uncover new dimensions of its function and regulation. Future research directions include:
- Structural Studies: High-resolution structural studies of actin and actin-associated proteins will provide deeper insights into their interactions and functions. Techniques such as cryo-electron tomography and X-ray crystallography will be instrumental in elucidating these structures.
- In Vivo Studies: Utilizing advanced imaging techniques, such as live-cell fluorescence microscopy and super-resolution microscopy, will enhance our understanding of actin dynamics in living cells and tissues.
- Drug Development: Targeting actin dynamics and interactions with specific inhibitors or modulators holds promise for developing therapeutic strategies for diseases related to cytoskeletal dysfunction.
- Systems Biology: Integrating data from various omics approaches will help in understanding the complex networks of actin and its interacting partners, providing a holistic view of its role in cellular processes.
In summary, actin is a versatile and essential protein involved in various cellular functions. Its dynamic nature and interactions with numerous proteins make it a central player in cell biology. Ongoing research into its structure, function, and regulation continues to provide valuable insights into both basic cellular mechanisms and the pathogenesis of actin-related diseases.
Integrin Signaling: A short Review – The Science Notes