What is Electron Transport chain?
The electron transport chain (ETC) is a sequence of redox reactions that take place in a cell’s inner membrane of the mitochondria (or plasma membrane in prokaryotes). The ETC is essential in the process of oxidative phosphorylation, which is the method by which cells make ATP by transferring electrons using the ETC and subsequently production of ATP by ATP synthase.
During the ETC, electrons are transmitted down a succession of protein complexes (complex I, II, III, and IV) and mobile electron carriers (such as coenzyme Q and cytochrome c) in a sequence of redox processes, causing a proton gradient across the membrane. This proton gradient is subsequently utilized to fuel the production of ATP by ATP synthase, which uses the gradient’s energy to convert ADP and inorganic phosphate into ATP.
The ETC is a highly controlled mechanism that needs the coordinated activity of numerous proteins, coenzymes, and prosthetic groups. Any interruption in this process can result in the accumulation of reactive oxygen species (ROS) and oxidative stress, which can damage cellular components and contribute to the development of a range of illnesses.
Components of Electron transport chain
The electron transport chain (ETC) is made up of protein complexes and electron carriers that are found in the inner mitochondrial membrane of eukaryotic cells or the plasma membrane of prokaryotic cells. The ETC consists of the following components:
- Complex I (NADH dehydrogenase): This complex takes in electrons from NADH and transfers them to ubiquinone (Q) while pumping protons across the inner mitochondrial membrane.
- Complex II (succinate dehydrogenase): This complex receives electrons from FADH2 (which is created during the citric acid cycle) and transfers them to ubiquinone, but it does not pump protons.
- Ubiquinone (coenzyme Q): Ubiquinone is a mobile electron carrier which accepts electrons from complexes I and II and transfers them to complex III.
- Complex III (cytochrome bc1 complex): Complex III accepts electrons from ubiquinone and transfers them to cytochrome c while pumping protons.
- Cytochrome c: This mobile electron carrier accepts electrons from complex III and passes them to complex IV.
- Complex IV (cytochrome c oxidase): This complex accepts electrons from cytochrome c and transfers them to oxygen, the final electron acceptor. The reduction of oxygen to water also contributes to the proton gradient.
The exchange of electrons between these components initiates a sequence of redox reactions that result in a proton gradient across the inner mitochondrial membrane. The ATP synthase enzyme uses this gradient to generate ATP from ADP and inorganic phosphate.
Steps of electron transport chain
The electron transport chain (ETC) is a sequence of redox reactions that take place in eukaryotic cells’ inner mitochondrial membranes or prokaryotic cells’ plasma membranes. The steps of ETC process are as follows:
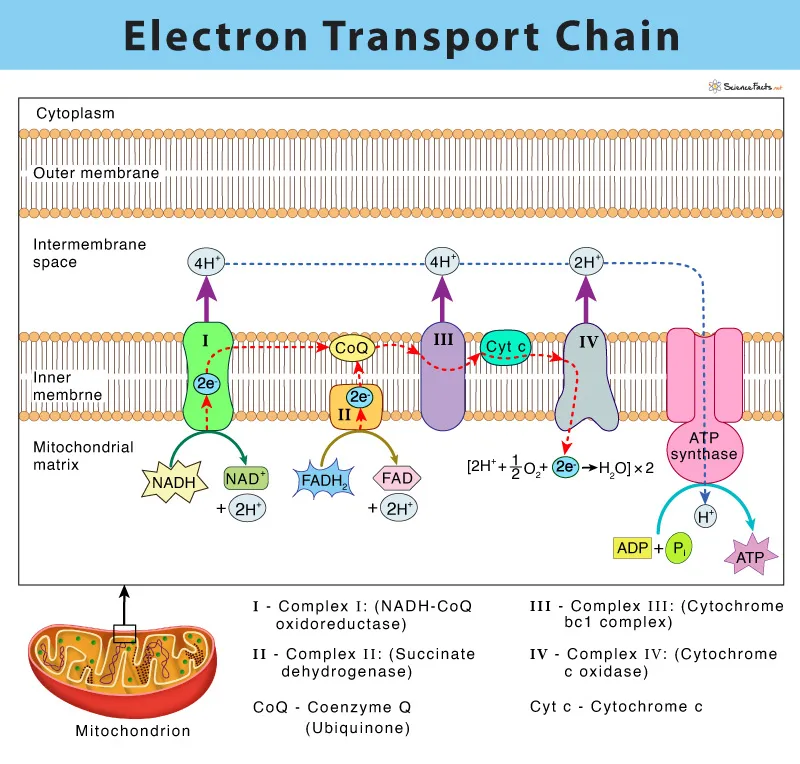
- NADH and FADH2, which are synthesized in early phases of cellular respiration such as glycolysis, pyruvate oxidation, and the citric acid cycle, provide electrons to the ETC.
- Complex I (NADH dehydrogenase) accept NADH electrons and converts them to ubiquinone (Q), a mobile electron carrier.
- Ubiquinone (Q) transports protons (H+) through the inner mitochondrial membrane while passing electrons to complex III (cytochrome bc1 complex).
- Complex III pumps’ protons across the membrane while transferring electrons to cytochrome c, another mobile electron carrier.
- Cytochrome c transmits electrons to complex IV (cytochrome c oxidase), which then transfers the electrons to oxygen (O2). The reduction of oxygen to water (H2O) contributes to the proton gradient across the inner mitochondrial membrane.
- Protons are pushed across the inner mitochondrial membrane when electrons travel through complexes I, III, and IV, generating a proton gradient.
- ATP synthase uses the energy stored in the proton gradient to synthesis ATP from ADP and inorganic phosphate (Pi) via a process known as oxidative phosphorylation.
ETC energy equation
The overall energy equation for the ETC can be represented as:
NADH + H+ + 1/2O2 + ADP + Pi -> NAD+ + H2O + ATP
where
- NADH is the electron donor,
- H+ is the proton,
- O2 is the electron acceptor,
- ADP is adenosine diphosphate,
- Pi is inorganic phosphate,
- NAD+ is the electron acceptor,
- H2O is the product of the final redox reaction,
- and ATP is the energy currency of the cell.
ETC energy calculation
The electron transport chain (ETC) is a set of redox reactions that take place in the inner mitochondrial membrane (or, in prokaryotes, the plasma membrane). The transport of electrons through the ETC creates a proton gradient across the membrane, which is utilized to drive ATP synthase.
The energy released during the transfer of electrons through the ETC can be calculated using the Gibbs free energy equation:
ΔG = -nFΔE
where,
- ΔG is the change in Gibbs free energy,
- n is the number of electrons transferred,
- F is Faraday’s constant (96,485 C/mol),
- and ΔE is the change in standard electrode potential (in volts) for the redox reaction.
The standard electrode potential for the NADH/NAD+ couple is -0.32 V, while that for the O2/H2O couple is 0.82 V.
The ΔE for the transfer of two electrons from NADH to O2 through the ETC is therefore:
ΔE = Eo,O2/H2O – Eo,NADH/NAD+ = 0.82 – (-0.32) = 1.14 V
Using this value in the Gibbs free energy equation, the energy released during the transfer of two electrons from NADH to O2 through the ETC can be calculated as:
ΔG = -2 × 96,485 × 1.14 = -220.5 kJ/mol
This value represents the amount of energy released per mole of electrons transferred through the ETC. This energy is harnessed by the proton gradient to drive ATP synthesis, which is used by cells to carry out a variety of biological processes.
ETC inhibitors
Inhibitors of the electron transport chain (ETC) are substances that disrupt the ETC’s normal activity, resulting in a reduction in ATP synthesis and perhaps cellular harm. ETC inhibitors include the following:
- Rotenone: a naturally occurring chemical found in some plants that inhibits complex I of the ETC by preventing electron transport from NADH to ubiquinone.
- Antimycin A: A Streptomyces strain-produced antibiotic that inhibits complex III of the ETC by attaching to the ubiquinone binding site.
- Cyanide and carbon monoxide: both chemicals inhibit complex IV of the ETC by attaching to the heme group in cytochrome c oxidase and preventing electron transport to oxygen.
- Oligomycin: an antibiotic generated by Streptomyces strains that inhibits ATP synthase by attaching to the F0 subunit, preventing protons from passing through the ATP synthase complex.
- Uncouplers: chemicals that disturb the proton gradient across the inner mitochondrial membrane, decoupling oxidative phosphorylation from ATP generation. 2,4-dinitrophenol (DNP) and carbonyl cyanide m-chlorophenyl hydrazone (CCCP) are examples of uncouplers.
ETC inhibitors can be important research tools for understanding the operation of the ETC and the control of cellular metabolism. They may, however, be poisonous and detrimental to cells and organisms, and in some cases, they can even be utilized as poisons.
Importance of Electron transport chain
The electron transport chain (ETC) is vital for the generation of ATP, the cell’s principal energy currency. Here are some of the ETC’s main responsibilities and functions:
- ATP production: In prokaryotes, the ETC creates a proton gradient across the inner mitochondrial membrane or the plasma membrane. The ATP synthase enzyme uses this proton gradient to create ATP by oxidative phosphorylation from ADP and inorganic phosphate (Pi). This is the most efficient route for the cell to create ATP, supplying a significant quantity of energy for metabolic activities.
- Redox reactions: The ETC is made up of a series of redox reactions that transport electrons from electron donors to electron acceptors (such as oxygen). These redox reactions are accompanied by proton pumping across the membrane, resulting in a proton gradient. This gradient allows for the spontaneous flow of electrons, releasing energy that may be used to make ATP.
- Metabolic regulation: The ETC can govern cellular metabolism by regulating the availability of reducing equivalents (electrons) in the form of NADH and FADH2. The ETC can also control the synthesis of reactive oxygen species (ROS), which are toxic byproducts of aerobic metabolism. To prevent cellular damage, the ETC can assist maintain the equilibrium between ROS generation and clearance.
- Mitochondrial function: The ETC is found within the inner mitochondrial membrane, which regulates the transport of chemicals into and out of the mitochondria. The ETC also contributes to the maintenance of mitochondrial membrane potential, which is required for the normal activity of mitochondrial enzymes and transporters.
In summary, the electron transport chain is an important aspect of cellular respiration because it allows for efficient ATP synthesis while also controlling cellular metabolism and sustaining mitochondrial function.