History of Aminoglycosides
Aminoglycosides are potent, broad-spectrum antibiotics that work by inhibiting protein synthesis. Since streptomycin was first discovered from Streptomyces griseus and adopted for medical use in 1944, Aminoglycosides has been an essential component of antibacterial chemotherapy.
Streptomycin, the first aminoglycoside to be identified, was extracted from Streptomyces griseus by Selman Waksman and his group at Rutgers University in 1943. The 1950s and 1960s observed widespread usage of streptomycin as a treatment for tuberculosis since it was the first antibiotic proven to be effective against the illness.
Over the years, new members of the class have been introduced, which includes neomycin (1949, S. fradiae), kanamycin (1957, S. kanamyceticus), gentamicin (1963, Micromonospora purpurea), netilmicin (1967, derived from sisomicin), tobramycin (1967, S. tenebrarius), and amikacin (1972, derived from kanamycin). These antibiotics are used to treat a range of bacterial illnesses, among which are brought on by Gram-negative bacteria like Escherichia coli and Pseudomonas aeruginosa. Each of these antibiotics exhibits a slightly different spectrum of efficacy.
The 1980s saw a trend away from systemic usage of the class when third-generation cephalosporins, carbapenems, and fluoroquinolones were available and believed to be less toxic and/or offer greater coverage compared to aminoglycosides. However, rising resistance to these drug classes and greater understanding of the causes of aminoglycoside resistance have reestablished interest in legacy aminoglycosides and the creation of novel aminoglycosides.
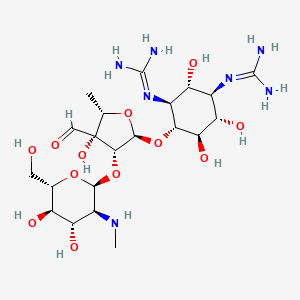
Antimicrobial activity of Aminoglycosides
Aminoglycosides are a structurally distinct family of natural antibiotics that are nowadays accessible for the treatment of bacterial infections. Aminoglycosides are classified as cationic compounds because of the many amino groups they contain.
Numerous Gram-negative and some Gram-positive bacteria are susceptible to the broad-spectrum antimicrobial activity of aminoglycosides. Acinetobacter baumannii, Stenotrophomonas maltophilia, and other aerobic, non-fermenting bacteria are particularly susceptible to their effects. Some mycobacteria, such as Mycobacterium tuberculosis, are also susceptible to aminoglycoside treatment.
Aminoglycosides’ capacity to attach to the bacterial ribosome to hinder protein synthesis is the basis for their antibacterial impact. They attach to the ribosome’s 30s subunit and stop the genetic code from being correctly read, which results in the synthesis of aberrant proteins and ultimately causes bacterial death.
They have a bactericidal effect that is concentration-dependent, meaning that larger concentrations of the medication result in faster bacterial eradication. They frequently work better against specific bacterial illnesses when combined with other antibiotics like beta-lactams or vancomycin.
Aminoglycosides are fundamentally inactive against anaerobic bacteria because active electron transport is necessary for aminoglycoside absorption into cells. Additionally, most Burkholderia spp., Stenotrophomonas spp., Streptococcus spp., and Enterococcus spp. are unaffected by aminoglycosides.
Structure of Aminoglycosides
Aminoglycosides are a class of antibiotics that are composed of amino sugars and an aminocyclitol ring. The structure of aminoglycosides can vary depending on the specific drug, but they all share some common structural features. Here are some key structural characteristics of aminoglycosides:
- Aminosugars: It contain one or more amino sugars, such as streptomycin’s D-streptamine.
- Aminocyclitol ring: Aminoglycosides also contain an aminocyclitol ring, such as neomycin’s neamine ring.
- Amino groups: They have multiple amino groups, which give them their basic properties.
- Glycosidic linkages: They are typically linked together by glycosidic linkages between the various sugar molecules.
- Substituents: Different aminoglycosides can have different substituents attached to the sugar and aminocyclitol rings, which can affect their pharmacological properties.
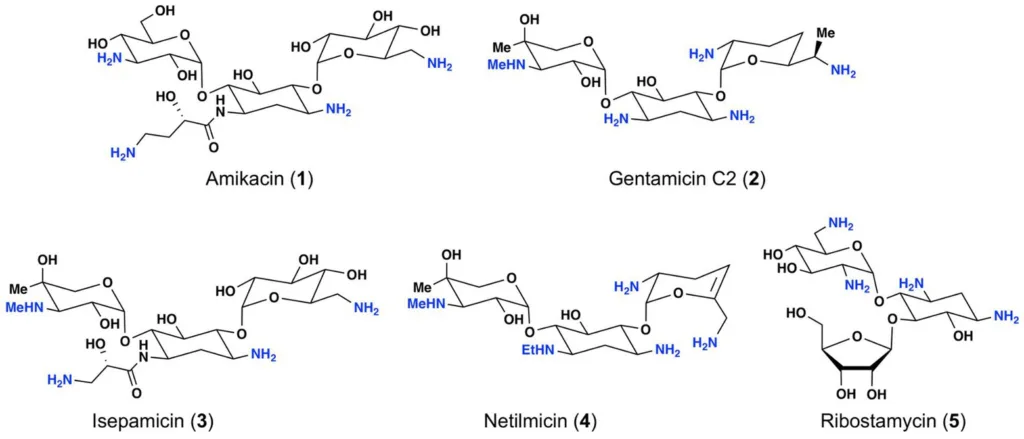
Mechanism of Action
- Aminoglycosides link with great affinity, irreversibly to the A-site on the 30s ribosome’s 16s ribosomal RNA to inhibit protein synthesis.
- All members of the aminoglycoside class modify the conformation of the A-site, while having varying specificities for various A-site regions.
- As an outcome of this interaction, the antibiotic stimulates genetic codon misreading upon delivery of the aminoacyl transfer RNA, which in turn causes mistranslation.
- This leads to error-prone protein synthesis, which enables the assembly of the wrong amino acids to a polypeptide and subsequent release to harm the cell membrane and other tissues.
- These mistranslated proteins enter the cytoplasmic membrane, insert there, damage the membrane, and then make it easier for aminoglycosides to enter.
- It also causes the cytoplasm to rapidly absorb more aminoglycoside units.
- They also disrupt the ribosome’s ability to proofread the errors, which results in more mistakes being made during the synthesis of proteins.
- This causes an increase in the inhibition of protein synthesis, mistranslation, and speedy cell death
- Aminoglycosides have a concentration-dependent bactericidal action, which means that bacterial death is increased with increasing medication concentrations.
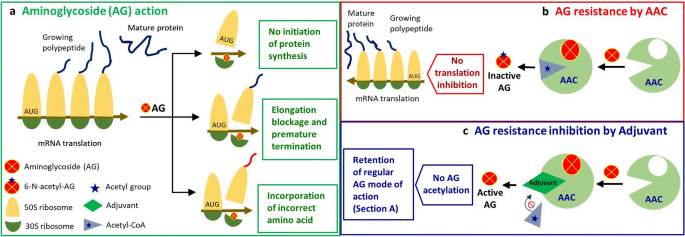
Mechanisms of Aminoglycoside Resistance
There are numerous distinct ways that aminoglycoside resistance emerges, including enzymatic modification, target site alteration by an enzyme or chromosomal mutation, and efflux.
Enzymatic Drug Modification:
Aminoglycosides modifying enzymes (AMEs) reduce the antibiotic’s affinity for binding to its target, decreasing the antibacterial efficacy of the antibiotic.
- Aminoglycoside acetyltransferases acetylate amino groups found at different positions on the aminoglycoside scaffold in an acetyl-CoA-dependent reaction.
- Aminoglycoside phosphotransferases catalyze the ATP-dependent phosphorylation on aminoglycoside with hydroxyl groups. These enzymes reduce the affinity for binding to the target by reducing the hydrogen bonding potential between aminoglycoside hydroxyl groups with key rRNA residues.
- Aminoglycoside nucleotidyltransferases function by transferring AMP from an ATP donor to hydroxyl groups at positions 2′′, 3′′, 4′, 6, and 9.
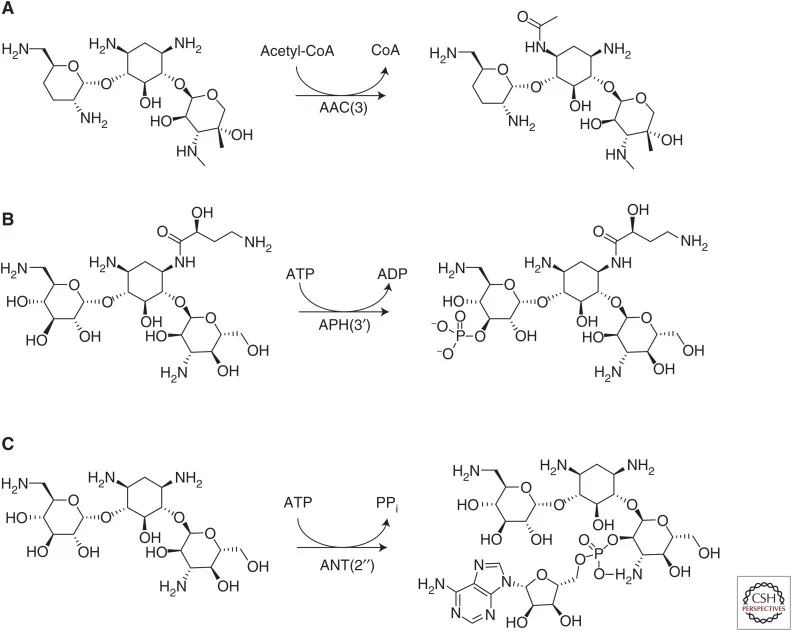
Target site modification:
The activity of 16s rRNA methyltransferases (RMTs) results in target site alteration, which leads to aminoglycoside resistance. These enzymes alter particular rRNA nucleotide residues, preventing aminoglycosides from attaching to the target.
Efflux-Mediated Resistance:
Bacteria with efflux-mediated resistance to aminoglycosides over express efflux pumps that are capable of pumping the drug out of the cell. As a result, the antibiotic has a lower concentration inside the bacterial cell, making it less efficient at blocking protein production and killing the bacterium.
The AcrAB-TolC pump in Escherichia coli and the MexXY-OprM pump in Pseudomonas aeruginosa are two examples of efflux pumps that can confer resistance to aminoglycosides. Because these pumps can also pump out other classes of antibiotics, efflux-mediated resistance is frequently associated with multidrug resistance.
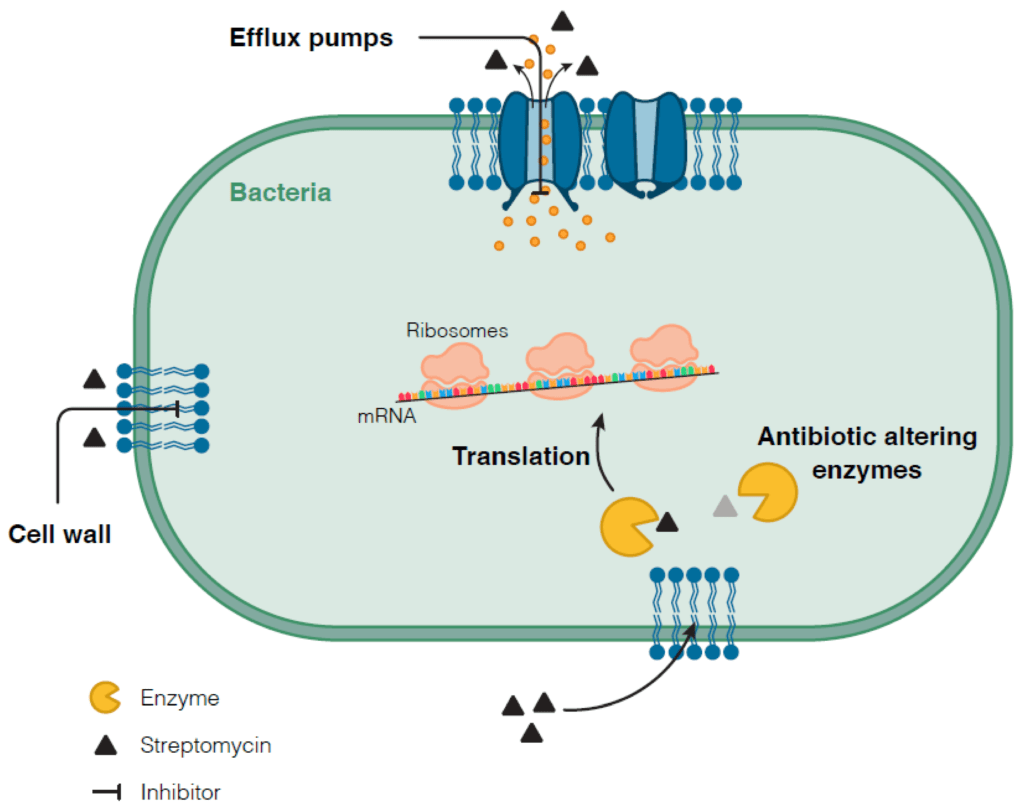
Measures to overcome Aminoglycoside Resistance
There are several strategies to overcome aminoglycoside resistance, some of which are:
- Combination therapy: Aminoglycosides can be used with other antibiotics like beta-lactams or fluoroquinolones to produce synergistic efficacy against certain bacterial infections.
- Modification of the drug: Alteration to the structure of the aminoglycoside molecule can increase its efficiency while decreasing vulnerability to resistance mechanisms. Liposomal preparations of aminoglycosides, for example, have been found to boost their absorption into bacterial cells and effectiveness against resistant strains.
- Use of alternative drugs: Alternative drugs may be used to treat bacterial infections when aminoglycoside resistance is present. Tetracyclines, macrolides, and fluoroquinolones, for example, are frequently effective against many of the same bacteria that aminoglycosides target.
- Inhibition of resistance mechanisms: Efflux pumps, which are one of the main mechanisms of aminoglycoside resistance, can be targeted with efflux pump inhibitors. These inhibitors can block the activity of the efflux pump and increase the concentration of the drug inside the bacterial cell.
- Combination of aminoglycosides with non-antibiotic compounds: Studies have shown that combining aminoglycosides with non-antibiotic compounds, such as EDTA or silver nanoparticles, can enhance their antibacterial activity against resistant strains.