Epigenetics
Early in the 1940s, Conrad Waddington coined the word “epigenetics.” Epigenetics, according to him, is “the branch of biology which studies the causal interactions between genes and their products which bring the phenotype into being.” Epigenetics and gene expression will be described in detail here.
“Epi-“means on or above in Greek, and “epigenetic” describes factors beyond the genetic code. The field of epigenetics investigates how cells regulate gene function without altering the DNA sequence. Epigenetic alterations to DNA control whether or not genes are activated. These alterations are affixed to DNA and do not alter the order in which the DNA building units are arranged.
Epigenetics (also referred to as epigenomics) aims to understanding DNA modifications that do not require changes to the underlying sequence. The degree to which genes are switched on and off can be altered chemically in the DNA letters and proteins that associate with DNA. During cell division or from one generation to the next, specific epigenetic changes may be transferred from parent cell to daughter cell. An epigenome is a compilation of all epigenetic alterations in a genome.
Epigenetic modification patterns range between people, between tissues within an individual, and even between individual cells within a tissue. The epigenome can be impacted by environmental factors like a person’s nutrition and exposure to toxins. When cells divide epigenetic modifications can be preserved from cell to cell and, in some instances, passed down through the generations. Although reversible epigenetic changes can withstand numerous rounds of cell reproduction, they rarely last for generations in people.
Epigenetics has been defined and today is generally accepted as ‘‘the study of changes in gene function that are mitotically and/or meiotically heritable and that do not entail a change in DNA sequence.
Types of epigenetic changes
Gene expression is impacted by epigenetic modifications in a variety of ways. The following are the types of epigenetic changes:
DNA Methylation
DNA methylation is a regulatory process in which specific DNA regions are bound by a molecular substance called a methyl group (each consisting of one carbon atom and three hydrogen atoms) or hydroxymethyl group. By altering the DNA’s structure, this interaction affects how active or inactive genes are in producing proteins.
The methylation of the C5 position on cytosine bases, also known as m5c, is the change that is most frequently investigated. Using chromatin immunoprecipitation (ChIP) or bisulfite-based techniques, DNA methylation can be quantified.
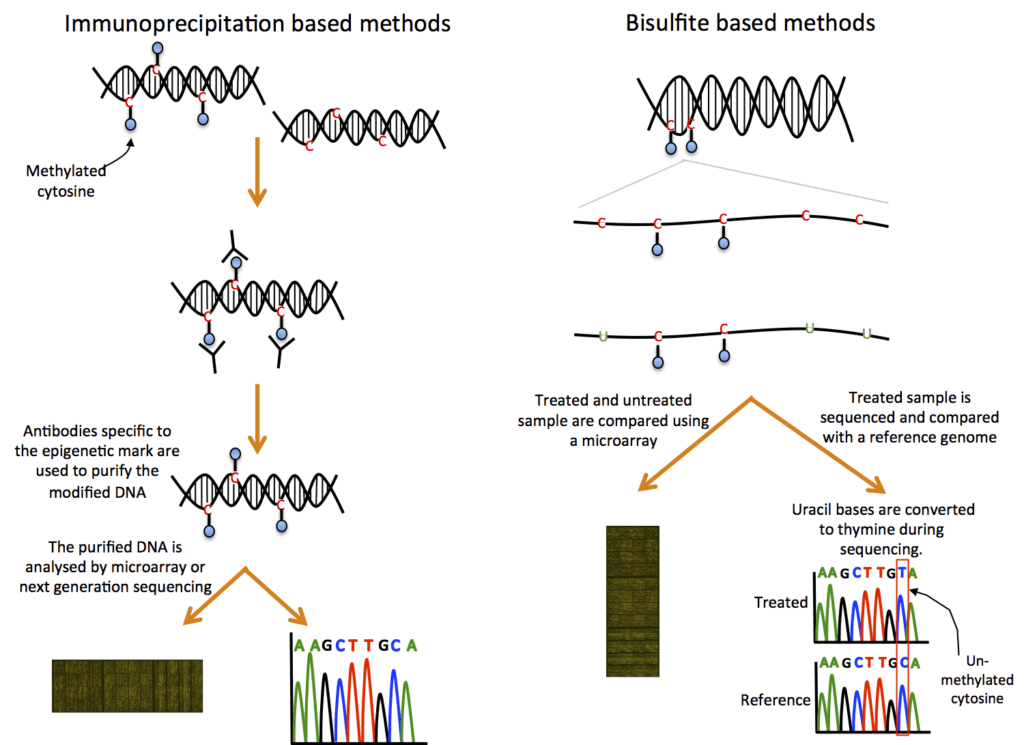
Histone modification
In the cell nucleus, histones serve as structural proteins. Histones are encircled by DNA, which gives chromosomes their structure. Histones can change by having molecular groups like methyl or acetyl groups (each consisting of two carbon, three hydrogen, and one oxygen atoms) added to them or removed from them. Chemical groups have an impact on how closely DNA is wound around histones, which impacts whether a gene can be activated or inactivated.
Histones can be altered in a variety of ways, typically at the N-terminal (or “tail”) end of the protein. These changes include acetylation, methylation, ubiquitination, citrullination, and phosphorylation of particular amino acids. By altering how histones attach to DNA, these changes have the ability to control gene expression in both positive and negative ways.
Numerous methods, such as mass spectrometry and genomic tools like ChIP-chip and ChIP-seq, can be used to identify histone changes. The genomics methods use chromatin immunoprecipitation (ChIP) of particular, modified histones and the DNA linked to them along with microarray (chip) or NGS (seq) of the DNA molecules to find areas of the genome linked to these changes.
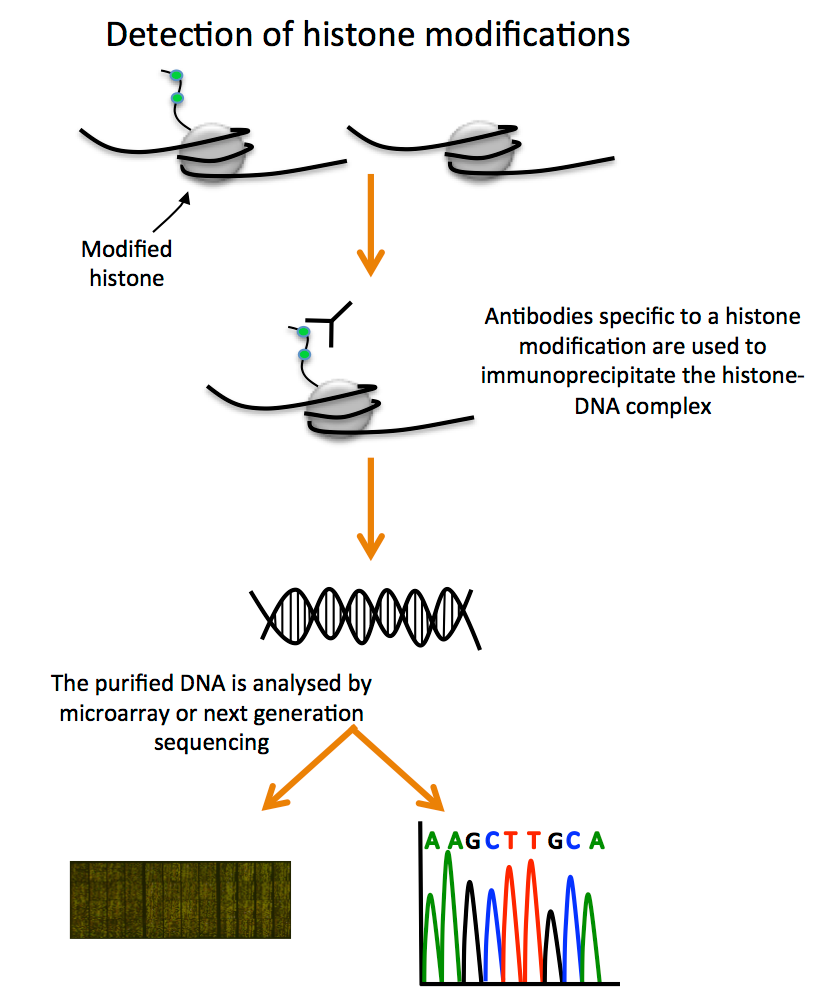
Non-coding RNA
Coding and non-coding RNA are created using DNA as the blueprint. Making proteins requires coding RNA. In order to prevent coding RNA from being used to create proteins, non-coding RNA attaches to it with some proteins. This breaks down the coding RNA. Additionally, non-coding RNA may enlist proteins to alter histones in order to “turn on” or “turn off” genes.
Genomic imprinting
Most genes have two functional versions that we receive from both our parents, one from each parent. With imprinted genes, however, we receive just one functional duplicate. Either the duplicate from mom or the copy from dad is epigenetically suppressed, depending on the gene. Typically, the addition of methyl groups during egg or sperm development is what silences an organism.
Imprinted genes typically retain their epigenetic markings for the duration of the body. However, they are restored when the egg and sperm are formed. Certain genes are always silenced in the egg, while others are always silent in the sperm, whether they originated from mom or dad.
Nutrition & the Epigenetics
Diet is one of the environmental variables in epigenetic change that is easier to study and, consequently, better known than behavior or stress.
When we take nutrients from food, they move through metabolic processes and are changed into compounds the body can use. One of these pathways produces methyl groups, significant epigenetic markers that inhibit genes.
Key elements of this methyl-making route include well-known nutrients like folic acid, B vitamins, and SAM-e (S-Adenosyl methionine, a common over-the-counter supplement). Diets rich in these methyl-donating foods can quickly change how genes are expressed, particularly in the early stages of development when the epigenome is still developing.
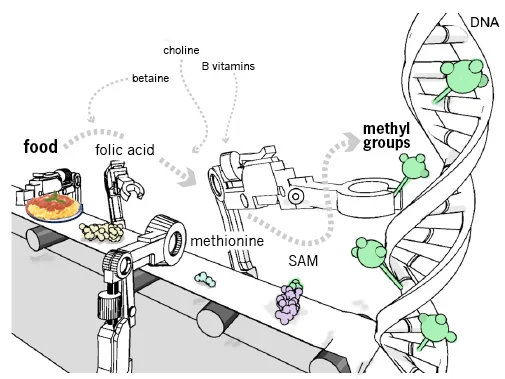
https://learn.genetics.utah.edu/content/epigenetics/nutrition
The epigenome can be impacted in ways that persist into maturity by the mother’s nutrition both during pregnancy and when you are a baby. Studies on animals have demonstrated that under-methylation of some areas of the genome can result from a diet that contains insufficient amounts of methyl-donating folate or choline before or shortly after birth.
DNA methylation declines in people who consume diets low in methyl, but these alterations can be reversed by reintroducing methyl to the diet.
Epigenetic errors
Abnormal gene activity or dormancy may result from errors in the epigenetic process, such as the alteration of the incorrect gene or the failure to add a chemical group to a specific gene or histone.
Depletion of DNA methylation appears to inhibit carcinogenesis if it is fueled by cancer suppressor hypermethylation. Depletion of DNA methylation increases cancer if it is caused by genomic instability.
In addition, histones can change in appearance in cancer, be mutated, disrupt chromosomal integrity, and cause other negative effects.
Genetic diseases are frequently brought on by altered gene activity, including that brought on by epigenetic mistakes. Epigenetic mistakes have been linked to diseases like cancer, biochemical issues, and degenerative illnesses.
The connection between the DNA and the substances that alter it is still being studied by scientists. They are focusing on how gene expression, protein synthesis, and human health are affected by epigenetic changes and errors.
References
- https://www.cdc.gov/genomics/disease/epigenetics.htm
- https://medlineplus.gov/genetics/understanding/howgeneswork/epigenome/
- https://www.genome.gov/genetics-glossary/Epigenetics
- https://www.ncbi.nlm.nih.gov/pmc/articles/PMC2791696/
- https://learn.genetics.utah.edu/content/epigenetics/
- https://www.ncbi.nlm.nih.gov/books/NBK532999/
- https://cs.stanford.edu/people/karpathy/EpigeneticsCourseranotes.pdf