Cellular respiration is the mechanism by which cells turn nutrients into ATP energy (adenosine triphosphate). It is a fundamental metabolic way found in all living creatures, ranging from single-celled bacteria to complex multicellular animals such as humans. In this article, we will look in depth at the process of cellular respiration (Krebs cycle), including its various stages, mechanics, and significance in maintaining cellular homeostasis.
Cellular respiration is divided into three stages: glycolysis, the Krebs cycle (also known as the citric acid cycle or TCA cycle), and oxidative phosphorylation. (Also known as the electron transport chain or ETC). These stages occur in the mitochondria of eukaryotic cells and the cytoplasm of prokaryotic cells, and they are interrelated and interdependent, with each stage relying on the products of the preceding one.
Glycolysis
Glycolysis occurs in the cytoplasm of cells and is the first stage of cellular respiration. It is a ten-step enzymatic process that converts one molecule of glucose (a six-carbon sugar) into two molecules of pyruvate. (a three-carbon molecule). Two molecules of ATP are used during glycolysis, while four molecules of ATP are created, resulting in a net gain of two ATP molecules. Furthermore, two molecules of NADH are formed, which can be employed in the electron transport chain to make further ATP.
Learn more:
Krebs cycle/ Citric Acid Cycle/ TCA cycle
The Krebs cycle is the second step of cellular respiration and takes place in cells’ mitochondria. It is an eight-step enzymatic process that converts pyruvate into ATP, NADH, FADH2, and CO2. Pyruvate is transformed to acetyl-CoA during the Krebs cycle, which enters the cycle and performs a series of oxidative and decarboxylation processes. Per molecule of glucose, the Krebs cycle generates two molecules of ATP, six molecules of NADH, two molecules of FADH2, and two molecules of CO2.
Oxidative Phosphorylation
The final stage of cellular respiration is oxidative phosphorylation, which takes place in the inner mitochondrial membrane of cells. It is a set of electron transport processes that generate ATP by using the NADH and FADH2 produced during glycolysis and the Krebs cycle. Electrons are transferred via a series of electron carriers during oxidative phosphorylation, and the energy created during this process is used to drive protons (H+) across the inner mitochondrial membrane. The resultant proton gradient is utilized by the ATP synthase enzyme to create ATP from ADP and Pi. (Inorganic phosphate). The end products of oxidative phosphorylation are water and ATP, with each molecule of NADH producing three ATP molecules and each molecule of FADH2 producing two ATP molecules.
Krebs Cycle/TCA cycle/
Citric Acid cycle
- The Krebs cycle is also known as the citric acid cycle or the tricarboxylic acid (TCA) cycle; is a basic metabolic pathway that is vital to cellular respiration.
- It is named after Sir Hans Krebs, who received the Nobel Prize in Physiology or Medicine in 1953 for his pioneering work.
- The Krebs cycle is a sequence of eight enzymatic events that take place in the mitochondria of eukaryotic cells and the cytoplasm of prokaryotic cells.
- The cycle begins with the condensation of acetyl-CoA and oxaloacetate to create citrate, which is then followed by a sequence of oxidations, decarboxylations, and substrate-level phosphorylations that lead to in the synthesis of ATP, NADH, FADH2, and CO2.
- The Krebs cycle is a critical source of energy and reducing power for the cell, and it is required for all aerobic organisms to function properly.
Steps of the Krebs cycle
There are eight stages in the Krebs cycle, and a specific enzyme catalyzes each one of them. The steps are summarized below:
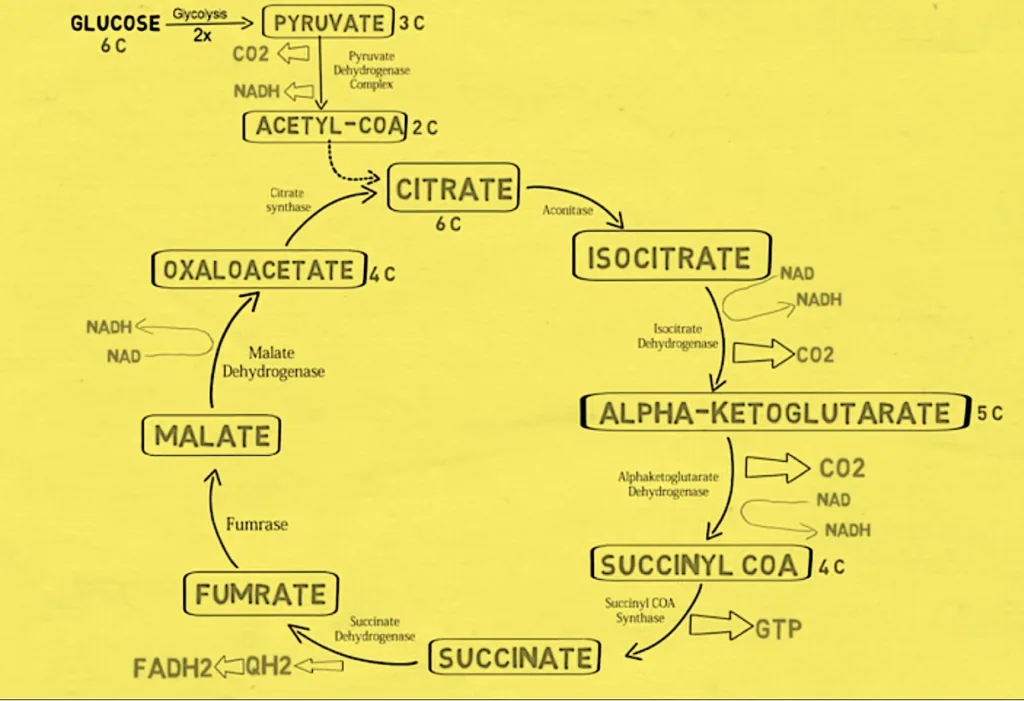
- Step 1
Citrate Synthesis
- Citrate is formed when acetyl-CoA reacts with oxaloacetate, a reaction catalyzed by citrate synthase.
The first step of the Krebs cycle begins when acetyl-CoA (2-Carbon molecule), which is derived from the breakdown of glucose, combines with oxaloacetate (4-Carbon molecule) to form citrate (6-Carbon molecule). This reaction is irreversible and is carried out by citrate synthase, an enzyme in mitochondria. The energy generated during this reaction is used to induce the formation of the high-energy thioester link between acetyl-CoA and oxaloacetate. The synthesis of citrate is an important phase in the Krebs cycle because it sets the stage for the rest of the cycle.
- Step 2
Isomerization of Citrate
- Citrate is converted to isocitrate by aconitase.
In the second step of the Krebs cycle, citrate is converted to isocitrate through the action of aconitase. This step involves the rearrangement of the citrate molecule to form isocitrate, a process that involves the removal and re-addition of a water molecule. This reaction is catalyzed by the enzyme aconitase and is reversible.
- Step 3
Oxidation of Isocitrate to Alpha-Ketoglutarate
- Isocitrate dehydrogenase converts isocitrate to alpha-ketoglutarate and generates NADH in the process.
In the third step of the Krebs cycle, isocitrate is oxidized to alpha-ketoglutarate (5-carbon molecule) by the enzyme isocitrate dehydrogenase. This step involves the removal of two electrons and two protons from isocitrate, which are transferred to NAD+ to produces NADH, which is an electron carrier that plays a crucial role in the electron transport chain. This step is an important source of energy for the cell, as it generates a high-energy intermediate in the form of alpha-ketoglutarate. This reaction is a key source of reducing power for the cell.
- Step 4
Conversion of Alpha-Ketoglutarate to Succinyl-CoA
- Alpha-ketoglutarate is converted to succinyl-CoA by alpha-ketoglutarate dehydrogenase, producing NADH and releasing CO2.
In the fourth step of the Krebs cycle, alpha-ketoglutarate is converted to succinyl-CoA, a four-carbon molecule, by the enzyme alpha-ketoglutarate dehydrogenase. This step involves the removal of two electrons and two protons from alpha-ketoglutarate, which are transferred to NAD+ to form NADH and releases CO2, which is a waste product. The production of succinyl-CoA is an important intermediate in the Krebs cycle, as it sets the stage for the production of ATP in the next step.
- Step 5
Conversion of Succinyl-CoA to Succinate
Succinyl-CoA is transformed to succinate by the activity of succinyl-CoA synthetase, which generates GTP in the process.
In the fifth phase of the Krebs cycle, the enzyme succinyl-CoA synthetase converts succinyl-CoA to succinate, a four-carbon molecule. This reaction involves the transfer of the high-energy thioester bond from succinyl-CoA to an ADP molecule, the formation of ATP, and the release of CoA.
- Step 6
Conversion of Succinate to Fumarate
- Succinate is oxidized to fumarate by the enzyme succinate dehydrogenase.
In the sixth stage of the Krebs cycle, succinate is oxidized to fumarate, a four-carbon molecule, by the enzyme succinate dehydrogenase. This process involves the transfer of two electrons and two protons to FAD, resulting in the formation of FADH2.
Succinate dehydrogenase is also known as complex II due to its position in the aerobic respiration chain. It catalyzes the reduction of ubiquinone to ubiquinol. The TCA cycle catalyzes the oxidation of succinate to fumarate, resulting in a decreased FADH2 from FAD.
- Step 7
Conversion of Fumarate to Malate
- Fumarate is hydrated to create malate, by the enzyme fumarase.
In the seventh phase of the Krebs cycle, fumarate is hydrated to create malate, a four-carbon molecule, by the enzyme fumarase. A molecule of water is added to fumarate during this process.
Fumarate catalyzes the reversible hydration of fumarate to malate (or fumarate hydratase). Another example of the interconnection of metabolic pathways is fumarate synthesis, which happens in the urea cycle.
- Step 8
Conversion of Malate to Oxaloacetate
- Oxidation of malate to oxaloacetate, by malate dehydrogenase.
In the final step OF TCA cycle’ Malate dehydrogenase is the is the enzyme responsible for the reversible oxidation of malate to oxaloacetate.
This enzyme is essential for NADH oxidation inside the TCA cycle. The delta-G-prime is positive, indicating that the reaction is favorable to malate. Citrate synthase, on the other hand, consumes oxaloacetate and forces this reaction forward to make additional oxaloacetate.
Cataplerotic Processes
Citric acid intermediates can exit the cycle and contribute to the formation of other molecules. Citrate can be directed toward fatty acids; succinyl-CoA can be directed toward heme synthesis; alpha-ketoglutarate can be directed toward amino acid synthesis, purine synthesis, and neurotransmitter production; oxaloacetate can be directed toward amino acid synthesis, and malate can be directed toward gluconeogenesis.
Anaplerotic Processes
Intermediates are introduced into the TCA cycle to replace cataplerotic activities and assure the cycle’s continuance. Pyruvate, for example, can enter the cycle throughout the body via pyruvate carboxylase, which adds oxaloacetate to the cycle. Because there is more oxaloacetate to participate in the reaction, the reaction is pushed ahead toward the already exergonic citrate synthase. Another example is the liver, which can create alpha-ketoglutarate through oxidative deamination or transamination of glutamate.
Functions of the Krebs cycle
1. Energy production:
The Krebs cycle, through the process of oxidative phosphorylation, is a key source of ATP, the primary energy currency of cells.
2. Reducing power generation:
The cycle generates reducing equivalents in the form of NADH and FADH2, which are then used to generate ATP in the electron transport chain.
3. Carbon dioxide production:
As a byproduct of the cycle, carbon dioxide is produced and expelled by respiration.
4. Biosynthesis of molecules:
The Krebs cycle intermediates can be employed as precursors for the production of amino acids, nucleotides, and other essential cellular components.
5. pH regulation:
The Krebs cycle contributes to cell pH regulation by creating and consuming protons during the processes.
6. Detoxification:
By transforming hazardous molecules like ammonia and other nitrogen-containing substances into less toxic forms, the cycle can detoxify them.
7. Metabolic integration:
The Krebs cycle is part of a wider metabolic network that includes glycolysis, fatty acid oxidation, and other pathways, and it is critical in integrating food metabolism.
Overall, the Krebs cycle is a complicated and highly regulated process that performs a variety of activities critical to cell survival and function.
Net output calculation of the Krebs cycle
Starting from one molecule of acetyl-CoA, the Krebs cycle produces the following net outputs:
- 2 molecules of CO2: produced during the decarboxylation reactions of isocitrate and alpha-ketoglutarate
- 3 molecules of NADH: produced during the oxidation of isocitrate, alpha-ketoglutarate, and malate
- 1 molecule of FADH2: produced during the oxidation of succinate
- 1 molecule of ATP (or GTP): produced during the substrate-level phosphorylation of GDP or ADP by succinyl-CoA synthetase
Note that the cycle also consumes one molecule of oxaloacetate, which is regenerated in the last step of the cycle by the conversion of malate to oxaloacetate. Therefore, the net output of the Krebs cycle from one molecule of acetyl-CoA is:
- 2 molecules of CO2
- 3 molecules of NADH
- 1 molecule of FADH2
- 1 molecule of ATP or GTP
These outputs are then used in the electron transport chain to generate ATP through oxidative phosphorylation.
Finally, the Krebs cycle, commonly known as the citric acid cycle, is essential for cellular respiration. This intricate chain of chemical processes transforms the stored energy in foods into ATP, the fundamental energy source for cells. Understanding the Krebs cycle is important in a variety of domains, including biology, biochemistry, and medicine. Despite its complexity, the Krebs cycle is a beautifully organized mechanism that happens in all living organisms, from bacteria to humans. Scientists can obtain insights into metabolic illnesses and generate novel therapeutic techniques by researching and dissecting the processes of the Krebs cycle.