RuBisCO stands for Ribulose-1,5-bisphosphate carboxylase/oxygenase.
Rubisco fixes atmospheric carbon dioxide into accessible sugar molecules.
Rubisco is an enzyme found in plant chloroplasts that aids in the fixation of carbon dioxide from the atmosphere during photosynthesis and the oxygenation of the resultant molecule during photorespiration.
- Rubisco, short for Ribulose-1,5-bisphosphate carboxylase/oxygenase, is the most abundant enzyme on Earth and is crucial for photosynthesis.
- It is found in autotrophic organisms like plants, bacteria, and archaea, and it facilitates the conversion of carbon dioxide (CO2) into organic compounds through the Calvin-Benson pathway.
- It is located in the mesophyll cells of C3 plants and the bundle sheath cells of C4 plants.
- It constitutes a significant portion of leaf protein and acts as a major nitrogen sink for plants.
- The estimated amount of Rubisco on Earth is approximately 5 kg per person.
- Rubisco functions as an enzyme by catalyzing the carboxylation of ribulose bisphosphate (RuBP), which is the primary CO2 acceptor in the Calvin cycle, initiating the process of carbon fixation.
- It displays both carboxylase and oxygenase activity, with the latter leading to photorespiration when molecular oxygen is used as a substrate.
Overall, Rubisco is a vital enzyme in the carbon fixation pathway, playing a crucial role in the photosynthetic processes of various organisms, including C3, C4, and CAM plants.
Discovery of RuBisCO
Rubisco, the most abundant enzyme on Earth, was first characterized by Sam Wildman in the 1950s. Spinach played a significant role in the discovery, as A.C. Chibnall, a British biochemist, separated the proteins of spinach leaves into two components shortly before World War II: a non-soluble and a soluble component.
- Its abundance in leaf chloroplasts of C3 plants, comprising 30-50% of proteins, and its high molecular weight of 550 kDa, led to the isolation of a homogeneous protein fraction known as “Fraction 1” through ammonium sulfate fractionation.
- The enzymatic function was initially unknown until researchers decoded the Benson-Bassham-Calvin Cycle and aimed to identify the enzyme involved in CO2 binding and the dismutation of 3-PGA.
- In 1956, the enzyme responsible for these processes was discovered and initially called carboxydismutase, but it was soon realized that it was the same protein as the previously identified “Fraction 1 protein,” known as Ribulose bisphosphate Carboxylase or RuBP Carboxylase.
- During the 1970s, it was further found that ribulose bisphosphate carboxylase also had the ability to bind oxygen.
- This bifunctional enzyme exhibited both carboxylase and oxygenase activities, leading to its name RubisCO (Ribulose bisphosphate Carboxylase Oxygenase).
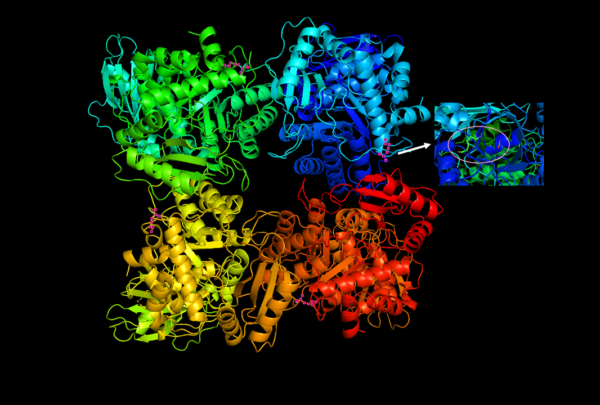
Structure of Rubisco
1. Subunit Composition:
Rubisco is composed of eight large subunits (L) weighing between 51 to 58 kDa each, and eight small subunits (S) weighing 12 to 18 kDa each, forming an L8S8 structure. The large subunits are encoded in the chloroplast genome and are produced in the chloroplast stroma, while the small subunits are encoded by the nuclear genome and imported into the chloroplast stroma through the outer and inner membranes of the chloroplast organelle. The assembly of these subunits into functional Rubisco involves chaperone proteins.
2. Catalytic and Regulatory Functions:
At the functional level, the large subunits of Rubisco contain the catalytic sites responsible for the enzyme’s activity in carbon fixation. On the other hand, the small subunits have a regulatory role in the enzyme’s function. The large chains form four dimers, while there are eight small chains. The large chains are encoded by chloroplast DNA, while the small chains are encoded by nuclear DNA and transported into the chloroplast stroma from the cytoplasm. Some organisms, such as certain bacteria and dinoflagellates, only possess the large subunits of Rubisco.
Characteristics of Rubisco
1. Abundance and Distribution:
Rubisco is the most abundant protein in the biosphere. It is present in various photosynthetic organisms, including algae, photosynthetic protists, plants, and autotrophic bacteria like cyanobacteria and proteobacteria. In C3 plants, Rubisco is predominantly found in mesophyll cells, while in C4 plants, it is present in bundle sheath cells. It accounts for approximately 50% and 30% of the total soluble leaf protein in C3 and C4 plants, respectively.
2. Activation and Active Site:
Rubisco requires magnesium ions (Mg2+) for its activity. It is inactive in the dark and gets activated in the light. Activation occurs through the binding of carbon dioxide (CO2) and Mg2+ ions to a lysine residue near the active site. This binding leads to conformational changes and stabilization of the active form of the enzyme. The active site of Rubisco is centered on a magnesium ion, which is tightly held by three amino acids: asparagine, glutamic acid, and a modified form of lysine. The binding of an activator carbon dioxide molecule to this lysine initiates the carbon fixation reaction. This activator carbon dioxide differs from the carbon dioxide molecule that is fixed to the sugar during photosynthesis.
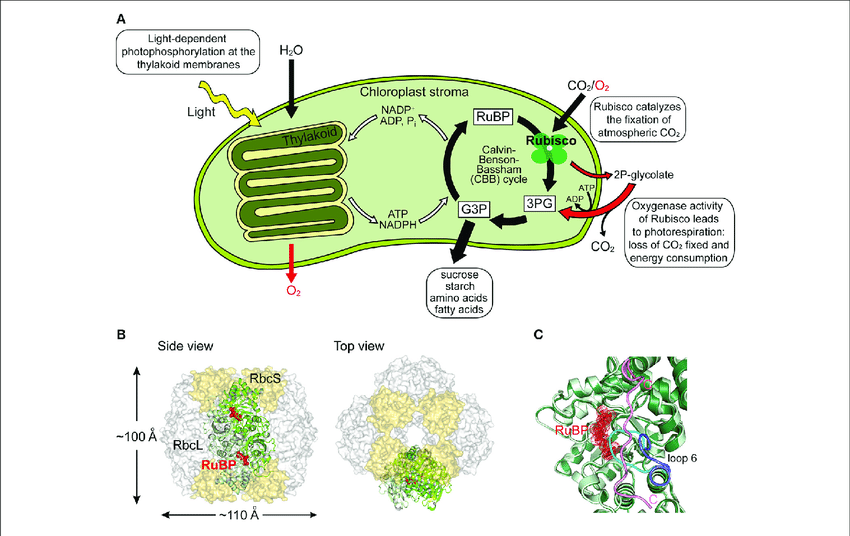
Functions of Rubisco
Role of Rubisco in Carbon Fixation:
The primary function of Rubisco is in photosynthesis, specifically in the carbon fixation process. It catalyzes the first step of carbon fixation in the C3 pathway or Calvin cycle by carboxylating Ribulose-1,5-bisphosphate (RuBP) with carbon dioxide (CO2) to form two molecules of 3-phosphoglyceric acid (3-PGA). This carboxylation reaction ensures the incorporation of CO2 into organic molecules, enabling the synthesis of sugars and other organic compounds.
Rubisco in Photorespiration:
Rubisco also plays a role in the process of photorespiration. Although it has a higher affinity for CO2, it can also bind to oxygen (O2). In conditions of high O2 and low CO2 concentrations, Rubisco exhibits oxygenase activity instead of carboxylase activity. This leads to the production of one molecule of phosphoglycerate (PGA) and one molecule of phosphoglycolate (P-glycolate). P-glycolate is subsequently converted to glycolate in a series of reactions known as photorespiration. Photorespiration can be seen as a wasteful process because it consumes energy and reduces the efficiency of carbon fixation.
Regulation and Competition:
Rubisco is subject to regulation by the concentrations of CO2 and O2. In the presence of high CO2 concentrations, Rubisco predominantly functions as a carboxylase, favoring the synthesis of PGA molecules and the continuation of the Calvin cycle. However, when O2 concentrations are high and CO2 concentrations are low, it exhibits oxygenase activity, leading to the production of PGA and P-glycolate through the oxygenation reaction. The competition between CO2 and O2 at the catalytic sites of Rubisco influences the balance between photosynthesis and photorespiration.
Rubisco as Limiting Factor:
Rubisco is known to be a relatively slow enzyme with a low turnover rate. This means that its catalytic efficiency is not very high, and it can become a limiting factor in photosynthesis, even under favorable conditions. The relatively low activity of this enzyme is attributed to mechanical and evolutionary reasons. It evolved in an oxygen-free atmosphere and later had to adapt to distinguish between CO2 and O2, resulting in a reduction in reaction speed to improve specificity.
Genetic Manipulation:
Due to its central role in carbon fixation, it has been a target for genetic manipulation to improve its catalytic properties. Researchers aim to enhance it’s efficiency, specifically in discriminating between CO2 and O2 and increasing carboxylation catalytic efficiency. However, the complex and large structure of Rubisco, as well as variations among different species, present challenges in identifying specific residues for improvement. Genetic engineering provides a promising approach to study the function and potential enhancement.
FAQs
Q1: What is Rubisco?
Rubisco, also known as Ribulose-1,5-bisphosphate carboxylase/oxygenase, converts atmospheric CO2 into organic molecules during photosynthesis. It is abundant and vital for carbon fixation.
Q2: What does Rubisco do?
It catalyzes the carboxylation of RuBP in the Calvin cycle, producing 3-PGA. This process fixes CO2 into organic compounds for further utilization.
Q3: How does Rubisco function?
It binds CO2, facilitating the carboxylation reaction to form 3-PGA. It can also bind oxygen, leading to an oxygenation reaction called photorespiration. This reduces carbon fixation efficiency and generates phosphoglycolate.
Q4: Where is Rubisco found?
It is present in various photosynthetic organisms, including plants, algae, and certain bacteria. In plants, it resides in chloroplasts of mesophyll cells (C3 plants) and bundle sheath cells (C4 plants).
Q5: Is Rubisco a slow enzyme?
Yes, it is relatively slow with a low turnover rate. Its limited catalytic efficiency can become a photosynthesis-limiting factor. Genetic engineering aims to enhance it’s efficiency.
Q6: What is Rubisco’s role in photorespiration?
Rubisco’s oxygen affinity leads to photorespiration, where it binds oxygen instead of CO2. This process results in phosphoglycolate production and decreases carbon fixation efficiency.
Q7: Can Rubisco be genetically modified?
Yes, Rubisco is a target for genetic manipulation to improve its catalytic properties. Researchers aim to enhance CO2 specificity and catalytic efficiency. However, identifying specific residues for improvement is challenging due to it’s complexity.
Q8: Why is Rubisco important?
It is crucial for life as it drives photosynthesis, converting sunlight and CO2 into energy-rich organic compounds. It fixes carbon from the atmosphere, supporting the food chain and providing energy for all organisms.
References
- Wildman, S. G. (1957). Ribulose-diphosphate: an essential factor in photosynthetic carbon dioxide assimilation. Nature, 180(4596), 803-804.
- Chibnall, A. C. (1939). Isolation of a soluble protein from green leaves. Nature, 144(3649), 967-967.
- Andersson, I., & Backlund, A. (2008). Structure and function of Rubisco. Plant Physiology and Biochemistry, 46(3), 275-291.
- Spreitzer, R. J., & Salvucci, M. E. (2002). Rubisco: structure, regulatory interactions, and possibilities for a better enzyme. Annual Review of Plant Biology, 53(1), 449-475.
- Parry, M. A., Keys, A. J., Madgwick, P. J., & Carmo-Silva, E. (2008). Rubisco regulation: a role for inhibitors. Journal of Experimental Botany, 59(7), 1569-1580.
- Whitney, S. M., & Andrews, T. J. (2001). The gene for the most abundant protein on Earth. Trends in Genetics, 17(11), 569-574.
- Andersson, I., & Backlund, A. (2008). Techniques for the study of ribulose-1,5-bisphosphate carboxylase/oxygenase. Photosynthesis Research Protocols, 297-312.
Learn more