Discover the biosynthetic pathways, chemical properties, and diverse applications of 4-hydroxybenzoate, a versatile compound renowned for its antimicrobial properties and preservative capabilities. Learn about its synthesis through methods such as the Kolbe-Schmitt reaction, esterification, hydroxylation, and microbial biosynthesis. Explore how 4-hydroxybenzoate is utilized in industries ranging from food and pharmaceuticals to cosmetics and materials science.
What is 4-hydroxybenzoate?
4-Hydroxybenzoate, also known as para-hydroxybenzoate or p-hydroxybenzoate, is an organic compound (C7H6O3) belonging to the benzoate class. Structurally, it consists of a benzene ring with a hydroxyl group attached at the para position (position 4). This white crystalline compound is sparingly soluble in water but soluble in organic solvents. Renowned for its antimicrobial properties, 4-hydroxybenzoate serves as a preservative, extending the shelf life of various products and preventing spoilage. It is also used as a building block for the synthesis of coenzymes, pigments, antioxidants, and pharmaceuticals.
Structure of 4-Hydroxybenzoate
The structure of 4-hydroxybenzoate, also known as para-hydroxybenzoate or p-hydroxybenzoate, can be represented as follows:
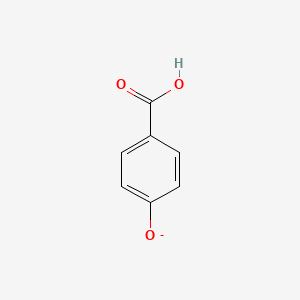
In this structure, the hydroxyl group (-OH) is attached to the benzene ring at the para position, which is the fourth carbon atom from the carboxyl group (-COOH) end. The carbon atoms in the benzene ring are numbered for reference.
The molecular formula of 4-hydroxybenzoate is C7H6O3, indicating that it consists of seven carbon atoms, six hydrogen atoms, and three oxygen atoms.
Physical Properties of 4-Hydroxybenzoate
- Physical State: 4-hydroxybenzoate is typically a white crystalline solid.
- Melting Point: The melting point of 4-hydroxybenzoate is around 213-215°C (415-419°F).
- Solubility: 4-hydroxybenzoate is sparingly soluble in water but more soluble in organic solvents.
- Odor: 4-hydroxybenzoate does not possess a strong characteristic odor.
- Molecular Weight: The molecular weight of 4-hydroxybenzoate is approximately 138.12 grams per mole.
Chemical Properties of 4-Hydroxybenzoate
- Acidic Nature: 4-hydroxybenzoate is a weak acid due to the presence of the carboxyl group (-COOH).
- Esterification: 4-hydroxybenzoate can undergo esterification reactions with alcohols in the presence of an acid catalyst.
- Hydroxylation: The hydroxyl group (-OH) in 4-hydroxybenzoate can undergo various reactions, including oxidation and substitution reactions.
- Reaction with Metals and Metal Salts: 4-hydroxybenzoate can form complexes with certain metals, such as aluminum, calcium, or iron.
- Reaction with Bases: 4-hydroxybenzoate can react with strong bases, such as sodium hydroxide, to form the corresponding salt.
- Polymerization: Under certain conditions, 4-hydroxybenzoate can undergo polymerization reactions, leading to the formation of polymers or copolymers with other monomers.
How is 4-hydroxybenzoate synthesized?
4-hydroxybenzoate, also known as para-hydroxybenzoate or p-hydroxybenzoate, can be synthesized through different methods depending on the desired application and starting materials available. Here are a few common synthetic routes for 4-hydroxybenzoate:
A. Kolbe-Schmitt Reaction:
The Kolbe-Schmitt reaction is a significant chemical reaction used for the synthesis of salicylic acid or its derivatives, including 4-hydroxybenzoate. It involves the transformation of phenol into salicylic acid by reacting with carbon dioxide in the presence of a strong base, typically sodium hydroxide. Here are the key steps involved in the Kolbe-Schmitt reaction:
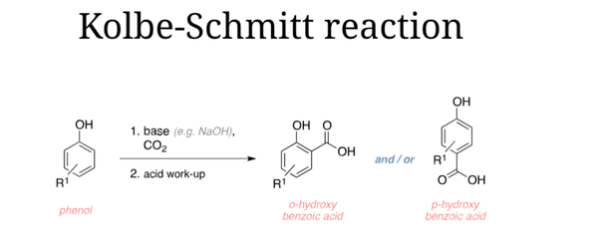
- Phenol Activation: Phenol (C6H5OH) is treated with a strong base, usually sodium hydroxide (NaOH), to form sodium phenoxide (C6H5ONa). This step is often carried out in an aqueous or alcoholic solution.
- Carbon Dioxide Addition: Carbon dioxide (CO2) is introduced to the reaction mixture containing sodium phenoxide. The CO2 reacts with sodium phenoxide to form sodium salicylate (C6H4(OH)CO2Na), an intermediate compound.
- Acidification: The sodium salicylate is then acidified, typically with the addition of a strong acid such as hydrochloric acid (HCl) or sulfuric acid (H2SO4). This acidification step leads to the precipitation of salicylic acid (C6H4(OH)COOH).
- Conversion to 4-Hydroxybenzoate: Salicylic acid can be further modified to obtain 4-hydroxybenzoate (C7H6O3), also known as para-hydroxybenzoate or p-hydroxybenzoate. This can be achieved through esterification, where salicylic acid reacts with an appropriate alcohol in the presence of an acid catalyst.
The Kolbe-Schmitt reaction is an important method for the industrial production of salicylic acid and its derivatives. It provides a practical route for obtaining 4-hydroxybenzoate, which finds applications in various industries, including food, pharmaceuticals, cosmetics, and materials science.
B. Esterification:
4-hydroxybenzoate can be synthesized through esterification of benzoic acid with an appropriate alcohol in the presence of an acid catalyst. For example, the reaction of benzoic acid with methanol in the presence of sulfuric acid as a catalyst would yield methyl 4-hydroxybenzoate (methylparaben).
C. Hydroxylation of Phenol:
Phenol can be hydroxylated to form 4-hydroxybenzoate using oxidizing agents such as hydrogen peroxide (H2O2) or peracetic acid (CH3CO3H) in the presence of a catalyst or enzyme. The reaction is typically carried out under controlled conditions to ensure selective hydroxylation at the para position of the benzene ring.
D. Microbial Biosynthesis
Certain microorganisms, such as bacteria and fungi, have the ability to produce 4-hydroxybenzoate through metabolic pathways. These pathways involve the enzymatic conversion of precursor molecules, such as shikimate or chorismate, into 4-hydroxybenzoate. The microbial biosynthesis of 4-hydroxybenzoate can be harnessed for industrial applications through fermentation processes.
It’s important to note that the specific synthetic method used for 4-hydroxybenzoate depends on the desired purity, scale of production, and availability of starting materials. Each method may have its advantages and limitations, and the choice of synthesis route will vary based on the specific requirements of the application.
4-Hydroxybenzoate Biosynthesis
The biosynthesis of 4-hydroxybenzoate refers to the natural production of this compound by living organisms. It occurs through various metabolic pathways depending on the organism and its metabolic capabilities. Here are some common biosynthetic routes for 4-hydroxybenzoate:
1. Shikimate Pathway:
The Shikimate pathway is not directly involved in the synthesis of 4-hydroxybenzoate (also known as para-hydroxybenzoate). 4-hydroxybenzoate is typically synthesized through other routes, such as the Kolbe-Schmitt reaction.
The Shikimate pathway primarily functions in the biosynthesis of aromatic amino acids and other aromatic compounds. However, it indirectly contributes to the production of 4-hydroxybenzoate through the following steps:
- Phenylalanine Biosynthesis: Within the Shikimate pathway, phenylalanine is one of the key aromatic amino acids synthesized. Phenylalanine serves as a precursor for the synthesis of 4-hydroxybenzoate.
- Phenylalanine Conversion: Phenylalanine can undergo enzymatic hydroxylation to form tyrosine. Tyrosine, in turn, can be converted into 4-hydroxyphenylpyruvate through enzymatic reactions.
- 4-Hydroxyphenylpyruvate to 4-Hydroxybenzoate: 4-hydroxyphenylpyruvate is further transformed into 4-hydroxybenzoate through a series of enzymatic steps. This conversion involves decarboxylation and reduction reactions.
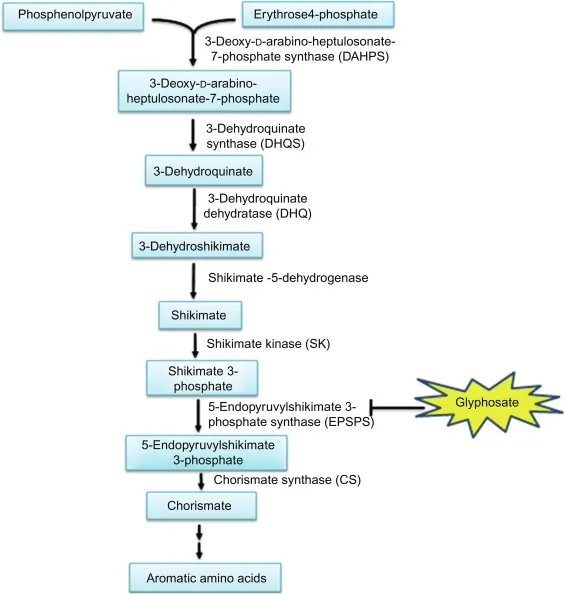
2. Degradation of Aromatic Compounds and 4-Hydroxybenzoate Production:
Some bacteria have developed metabolic pathways to degrade aromatic compounds like toluene or phenol. These pathways enable the breakdown of complex aromatic structures into simpler compounds, one of which is 4-hydroxybenzoate.
The degradation of aromatic compounds begins with the uptake of the target compound by the bacterial cell. Once inside, the bacterium initiates specific enzymatic reactions to metabolize the aromatic substrate.
- Initial Steps: The first step in the degradation process involves the conversion of the aromatic compound to an intermediate called catechol. This step is often carried out by enzymes known as dioxygenases, which incorporate molecular oxygen into the aromatic ring.
- Production of 4-Hydroxybenzoate: After the formation of catechol, further enzymatic reactions lead to the formation of 4-hydroxybenzoate. The exact pathway and enzymes involved may vary depending on the bacterial species.
- Enzymatic Diversity: Different bacteria may utilize distinct enzymes for aromatic compound degradation, resulting in variations in the specific degradation pathways. This diversity arises due to evolutionary adaptations, allowing bacteria to thrive in different environments and utilize a wide range of aromatic compounds as a carbon and energy source.
- Industrial Applications: The ability of certain bacteria to degrade aromatic compounds and produce 4-hydroxybenzoate has significant industrial applications. Bioremediation processes harness these bacteria to clean up environments contaminated with aromatic pollutants. Additionally, this microbial capability is leveraged in the production of 4-hydroxybenzoate as a valuable intermediate for various industrial processes.
3. Pathways Involving Aromatic Ring Hydroxylation:
Certain organisms, such as bacteria or fungi, possess specific enzymes capable of hydroxylating phenolic compounds, resulting in the synthesis of 4-hydroxybenzoate. These enzymes are commonly referred to as phenol hydroxylases or aromatic ring hydroxylases.
- Hydroxylation Process: Phenol hydroxylases catalyze the addition of a hydroxyl group (-OH) to the aromatic ring of precursor molecules. This enzymatic reaction leads to the formation of 4-hydroxybenzoate.
- Precursor Molecules: The precursor molecules involved in these pathways can vary, but they often include phenolic compounds such as phenol itself or other related aromatic substrates.
- Enzymatic Diversity: Different organisms possess distinct phenol hydroxylases, resulting in variations in the specific enzymatic pathways for aromatic ring hydroxylation. This diversity arises due to evolutionary adaptations and allows organisms to metabolize different phenolic compounds.
- Regulation and Expression: The expression of phenol hydroxylases is often regulated by the presence of phenolic compounds in the environment. When these compounds are available, the corresponding enzymes are upregulated, ensuring efficient hydroxylation and 4-hydroxybenzoate production.
- Industrial Applications: The ability of certain organisms to hydroxylate phenolic compounds and produce 4-hydroxybenzoate has practical applications. It can be harnessed in biotechnological processes to synthesize 4-hydroxybenzoate or related compounds of industrial significance.
- Biocatalysis and Green Chemistry: Enzymatic hydroxylation of aromatic compounds offers a more sustainable and environmentally friendly approach compared to traditional chemical synthesis methods. It enables the production of 4-hydroxybenzoate with high selectivity and efficiency, reducing the need for harsh chemicals and minimizing waste generation.
Applications of 4-Hydroxybenzoate
4-Hydroxybenzoate, also known as para-hydroxybenzoate or p-hydroxybenzoate, finds extensive applications across various industries. Here are some common applications of 4-hydroxybenzoate:
- Preservatives: 4-hydroxybenzoate and its derivatives, including methylparaben (methyl 4-hydroxybenzoate) and propylparaben (propyl 4-hydroxybenzoate), serve as widely used preservatives in food, beverages, pharmaceuticals, cosmetics, and personal care products. They effectively inhibit the growth of bacteria and fungi, extending the shelf life of these items.
- Pharmaceutical and Medical Uses: Methylparaben and propylparaben, which are derivatives of 4-hydroxybenzoate, are commonly employed as preservatives in pharmaceutical formulations to prevent microbial growth. They also function as excipients in drug formulations and exhibit antimicrobial properties in topical medications and ointments.
- Cosmetics and Personal Care Products: Methylparaben and propylparaben, among other derivatives of 4-hydroxybenzoate, are widely utilized as preservatives in cosmetics, skincare products, hair care products, and toiletries. Their usage helps thwart the growth of bacteria, yeasts, and molds, ensuring the safety and stability of these products.
- Industrial Applications: 4-hydroxybenzoate is employed as a primary material in the synthesis of various compounds in industrial processes. It acts as a precursor for the production of polymers like polyesters and polyurethanes. Moreover, derivatives of 4-hydroxybenzoate can serve as intermediates in the synthesis of dyes, pigments, antioxidants, and UV absorbers.
- Research and Laboratory Usage: 4-hydroxybenzoate and its derivatives are utilized in laboratory research, particularly in the fields of molecular biology and biochemistry. They function as components of buffers, staining solutions, and culture media for a variety of biochemical and cell-based experiments.
While 4-hydroxybenzoate and its derivatives possess diverse applications, it’s important to note that there are concerns about their safety and potential allergenic or sensitizing reactions in certain individuals. Regulatory bodies such as the FDA and EFSA have established guidelines to ensure the safe usage of these compounds in different products.
References
- Kim, T. W., Kim, H. U., & Zhang, Y. (2010). 4-Hydroxybenzoate/p-hydroxybenzoate degradation pathway in bacteria. Microbiology and Molecular Biology Reviews, 74(4), 524-534.
- Zhou, N. Y., Fuenmayor, S. L., & Williams, P. A. (2001). nag genes of Ralstonia (formerly Pseudomonas) sp. strain U2 encoding enzymes for gentisate catabolism. Journal of Bacteriology, 183(3), 700-708.
- Kohl, T. A., van der Rest, M. E., Frère, J. M., & Danchin, A. (1988). The last step in the biosynthesis of 4-hydroxybenzoate in Escherichia coli K-12: cloning, sequencing, and expression of the pabA gene. Journal of Bacteriology, 170(8), 3713-3720.
- Cao, H., Chen, X., & Zhou, N. (2014). Microbial biodegradation of chlorothalonil and its metabolite 4-hydroxybenzoate. Applied Microbiology and Biotechnology, 98(2), 835-843.
- Wang, C., Zhang, X., Chen, S., Zhang, C., Yang, X., Zhang, B., & Bi, Z. (2015). A novel para-hydroxybenzoate hydroxylase involved in the degradation of p-hydroxybenzoate in Comamonas testosteroni strain JH5. PLoS One, 10(4), e0124115.