In the always-changing field of molecular biology and genetic engineering, a revolutionary method called electroporation has taken center stage. This technique uses quick bursts of strong electric pulses to open cell membranes. This article explores electroporation in detail, explaining its basic rules, various uses, benefits, and challenges. It also emphasizes how this method has greatly influenced modern biotechnology and medical research.
Understanding Electroporation
Electroporation, also known as “electropermeabilization,” is an amazing technique in the world of molecular science. It works by using quick bursts of strong electric pulses to briefly open up cell walls. This temporary opening makes small holes that let things like DNA, RNA, medicines, and proteins go inside the cells. Originally, it was made for changing genes, but now it’s used in many different areas of science.
Principle of Electroporation:
- Electroporation relies on the application of electric fields to create transient pores in cell membranes.
- The electric pulses disrupt the lipid bilayer structure, leading to the formation of hydrophilic channels.
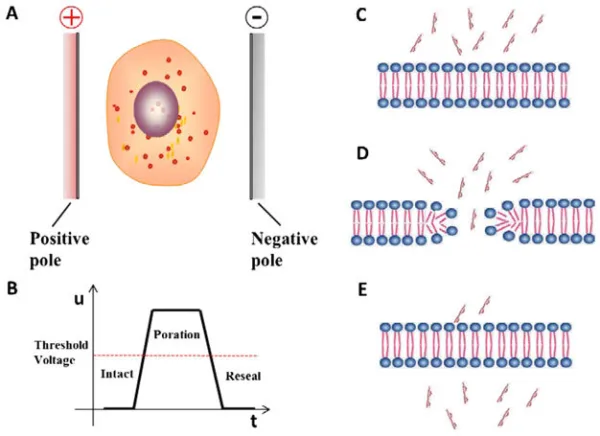
The Electroporation Process
The driving force behind electroporation lies in the interplay of electrophysics and cellular biology. By applying an external electric field, carefully calibrated to exceed the capacitance of the cell membrane, a reversible breakdown of the membrane occurs. This disruption triggers a state of transient permeability, during which molecules can traverse the destabilized membrane. This dynamic process, often likened to the opening of molecular gates, has proven instrumental in facilitating the introduction of foreign genetic material and other molecules into cells.
Methods of Electroporation
The electroporation is perfoemed through various methods, each tailored to specific experimental needs:
- Bulk Electroporation: This classic technique involves immersing a cell suspension within an electric field created by specialized cuvettes and electrodes. Efficient for transforming a multitude of cells, it finds utility in bacterial transformation and beyond.
- Microfluidic Electroporation: The advent of microfabricated devices has ushered in microfluidic technique allowing for precise control of electric fields and fluid dynamics. This innovation enables high-throughput and single-cell electroporation, elevating research possibilities.
- In Vivo Electroporation: Direct application to living tissues or organisms enables site-specific delivery of genetic material, proving indispensable in developmental biology and gene therapy endeavors.
General Electroporation Protocol
Electroporation is a powerful technique used to introduce foreign molecules, such as DNA, RNA, or proteins, into cells by creating temporary pores in the cell membrane through the application of electrical pulses. This protocol outlines a general procedure for performing the technique, which can be adapted for different cell types and applications.
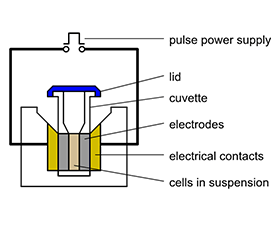
Materials:
- Cell culture medium
- Cells of interest
- DNA, RNA, or protein sample
- Buffer (e.g., phosphate-buffered saline, PBS)
- Cuvettes or plates
- Electroporator system (pulse generator)
- Pipettes and tips
- Incubator
- Cell culture dishes or plates
- Growth medium appropriate for your cells
Procedure:
- Cell Preparation:
- Culture cells in appropriate growth medium until they reach the desired confluency.
- Harvest cells by trypsinization or other suitable methods.
- Wash cells with electroporation buffer to remove growth medium and any residual trypsin.
- Sample Preparation:
- Prepare the DNA, RNA, or protein sample in a suitable buffer or solution. Concentration and volume should be optimized based on the application and cell type.
- Electroporation Setup:
- Set up the system according to the manufacturer’s instructions.
- Ensure that the cuvettes or plates are clean and dry.
- Electroporation Procedure:
- Mix the harvested cells with the prepared sample in a sterile tube. Gently mix by pipetting up and down.
- Transfer the cell-sample mixture to the cuvette or plate, ensuring no air bubbles are present.
- Pulse Parameters:
- Determine optimal pulse parameters (voltage, pulse duration, number of pulses) through optimization experiments. Start with lower settings and gradually increase if necessary.
- Ensure that the applied electrical pulses are appropriate for the cell type to avoid excessive damage.
- Electroporation:
- Place the cuvette or plate in the electroporator chamber.
- Apply the electrical pulses according to the chosen parameters.
- Recovery:
- Immediately transfer the electroporated cells from the cuvette or plate back to a sterile tube containing fresh culture medium.
- Allow cells to recover for a brief period (typically 10-30 minutes) at room temperature.
- Cell Culturing:
- Plate the recovered cells in appropriate culture dishes or plates containing growth medium.
- Incubate cells under suitable conditions (37°C, 5% CO2) for a specified period.
- Analysis and Applications:
- Depending on your experimental goals, analyze the electroporated cells for gene expression, protein production, or other desired outcomes.
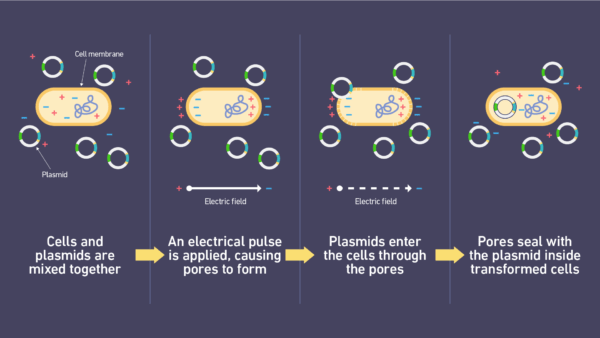
Notes:
- It is crucial to optimize the conditions for each specific cell type and application to achieve the highest transfection efficiency while maintaining cell viability.
- Always follow safety guidelines and manufacturer’s instructions when using electroporator equipment.
- Variation in protocols may be necessary based on cell type, sample, and research goals.
Applications of Electroporation
- Gene Delivery and Therapy:
- It enhances the uptake of foreign genetic material, aiding in gene therapy.
- Introduces therapeutic genes to treat genetic disorders like cystic fibrosis and muscular dystrophy.
- Cancer Treatment:
- Electrochemotherapy combines electroporation with chemotherapy to enhance drug uptake in cancer cells.
- Irreversible electroporation (IRE) targets and destroys tumor cells while preserving surrounding tissue.
- Bacterial Transformation:
- Facilitates the introduction of plasmid DNA into bacteria for genetic engineering.
- Cell-Based Assays:
- Enables efficient transfection of cells for research and drug development.
- Creates genetically modified cell lines for studying cellular processes.
- Stem Cell Engineering:
- Enhances differentiation and reprogramming of stem cells for regenerative medicine.
- Vaccine Production:
- It improves antigen delivery, enhancing immune responses in vaccine development.
Advantages of Electroporation
- Non-viral method, avoiding concerns of viral vector-associated risks.
- Versatile – works with a wide range of cell types and molecules.
- High transfection efficiency compared to other methods.
Challenges and Considerations:
- Cell viability may be compromised at high voltages or multiple pulses.
- Optimal parameters vary between cell types.
- Scalability for industrial applications.
Current Trends and Future Horizons:
- Nanopore Exploration: The creation of nanopores through electroporation opens avenues for DNA sequencing and single-molecule analysis, revolutionizing personalized genomics.
- Pulse Refinement: Ongoing efforts to fine-tune pulse parameters promise improved cell viability and heightened transfection efficiency, broadening the technique’s scope.
- Synergistic Delivery Techniques: Combining electroporation with nanotechnology and microfluidics introduces possibilities for precise and targeted therapeutic agent delivery.
Conclusion
Electroporation has left a lasting mark on molecular biology, playing a crucial role in understanding how cells talk to each other and driving significant progress in many different areas. Its special power to briefly open cell walls, letting in genetic material and molecules, has fueled important scientific findings and medical advancements. As we keep exploring and inventing new things in electroporation, its impact will always be a part of the ongoing story of scientific discovery, helping us uncover the secrets of life.
References
- Weaver JC. (1995). Electroporation: A General Phenomenon for Manipulating Cells and Tissues. Journal of Cellular Biochemistry, 51(4), 426-435.
- Neumann E., Schaefer-Ridder M., Wang Y., & Hofschneider P. H. (1982). Gene transfer into mouse lyoma cells by electroporation in high electric fields. The EMBO Journal, 1(7), 841-845.
- Heller R., & Heller L. C. (2006). Gene electrotransfer clinical trials. Advanced Genetics, 54, 83-98.
- Mir L. M., & Gehl J. (2010). Advances in electrochemotherapy, electrogene therapy, and use of electric pulses in general. European Journal of Cancer Supplements, 8(5), 42-49.
- Gehl J. (2003). Electroporation: theory and methods, perspectives for drug delivery, gene therapy and research. Acta Physiologica Scandinavica, 177(4), 437-447.
- Li S., Zhang X., Xia X., Zhou M., & Zhou Y. (2020). Electroporation: A novel application in cancer immunotherapy. Journal of Cancer, 11(16), 4796-4806.
- Miklavčič D., & Mali B. (2016). Handbook of Electroporation. Springer.
- Weaver J. C., Vaughan T. E., & Chizmadzhev Y. (1996). Theory of electrical creation of aqueous pathways across skin transport barriers. Advanced Drug Delivery Reviews, 18(3), 379-408.
Learn more